Geoscience Reference
In-Depth Information
an important concern is 'model drift' (a definite
tendency for the model climate to warm or cool
with time) due to accumulating errors from
the various component models. These tendencies
are often constrained by using observed clima-
tology at certain high-latitude or deep ocean
boundaries, or by adjusting the net fluxes of heat
and fresh water at each grid point on an annual
basis in order to maintain a stable climate, but
such arbitrary procedures are the subject of
controversy, especially for climate change studies.
Many important weather and climate
processes occur on a scale which is too small for
the typical GCM to simulate with a grid of several
degrees on a side. Examples of this would be the
radiative effects or latent heating due to cloud
formation or the transfer of water vapor to the
atmosphere by a single tree. Both processes greatly
affect our climate and must be represented for a
realistic climate simulation.
Parameterizations
are
methods designed to take into account the average
effect of cloud or vegetation process on an entire
grid cell. Parameterizations generally make use of
a statistical relationship between the large-scale
values calculated for the grid cell in order to
determine the effect of the parameterized process.
In order to gain confidence in the perfor-
mance of models in predicting future atmospheric
states, it is important to evaluate how well such
models perform in representing present-day
climate statistics. The Atmospheric Model Inter-
comparison Program (AMIP) is designed to do
this by comparing models from various centers
around the world using common procedures and
standardized data (on sea surface temperatures,
for example), as well as by providing extensive
documentation on the model design and the
details of model parameterizations. In this way
common deficencies can be detected and perhaps
attributed to a single process and then addressed
in future model versions.
Figure 8.3
compares
simulated zonally averaged surface temperature
for January and July for all AMIP participants
with the observed climatological mean. The
general features are well represented qualitatively,
Coupled model hierarchy
(A)
Atmospheric GCM
Swamp
SST from surface energy balance
(B)
Atmospheric GCM
Mixed lay
er
(slab)
SST from surface energy balance
heat storage
(C)
Atmospheric GCM
SST from surface
energy balance,
heat storage,
advection,
diffusion
Ocean
GCM
Figure 8.2
Schematic illustration of the three
types of coupling of an atmospheric GCM to the
ocean: (A) swamp ocean, (B) mixed layer, slab
ocean, (C) ocean GCM.
Source: From Meehl in Trenberth (1992) Copyright ©
Cambridge University Press.
dynamic ocean models which solve appropriate
equations for the ocean circulation and thermo-
dynamic state similar to 1-5 above and which are
coupled to atmospheric models. Such coupled
models are referred to as Atmopshere-Ocean
General Circulation Models (AOGCMs). When
the global ocean is considered, seasonal freezing/
melting and the effects of sea ice on energy
exchanges and salinity must also be modeled.
Therefore, dynamic sea ice models, which actively
calculate the thickness and extent of ice, are now
replacing the specification of climatological sea
ice. Because of the century-long timescale of deep
ocean circulations, the use of a dynamic ocean
model requires large amounts of simulation time
for the different model components to equilibrate
which greatly increases the cost of running these
models.
Because coupled AOGCMs are used in long-
term (century or millennium scale) simulations,
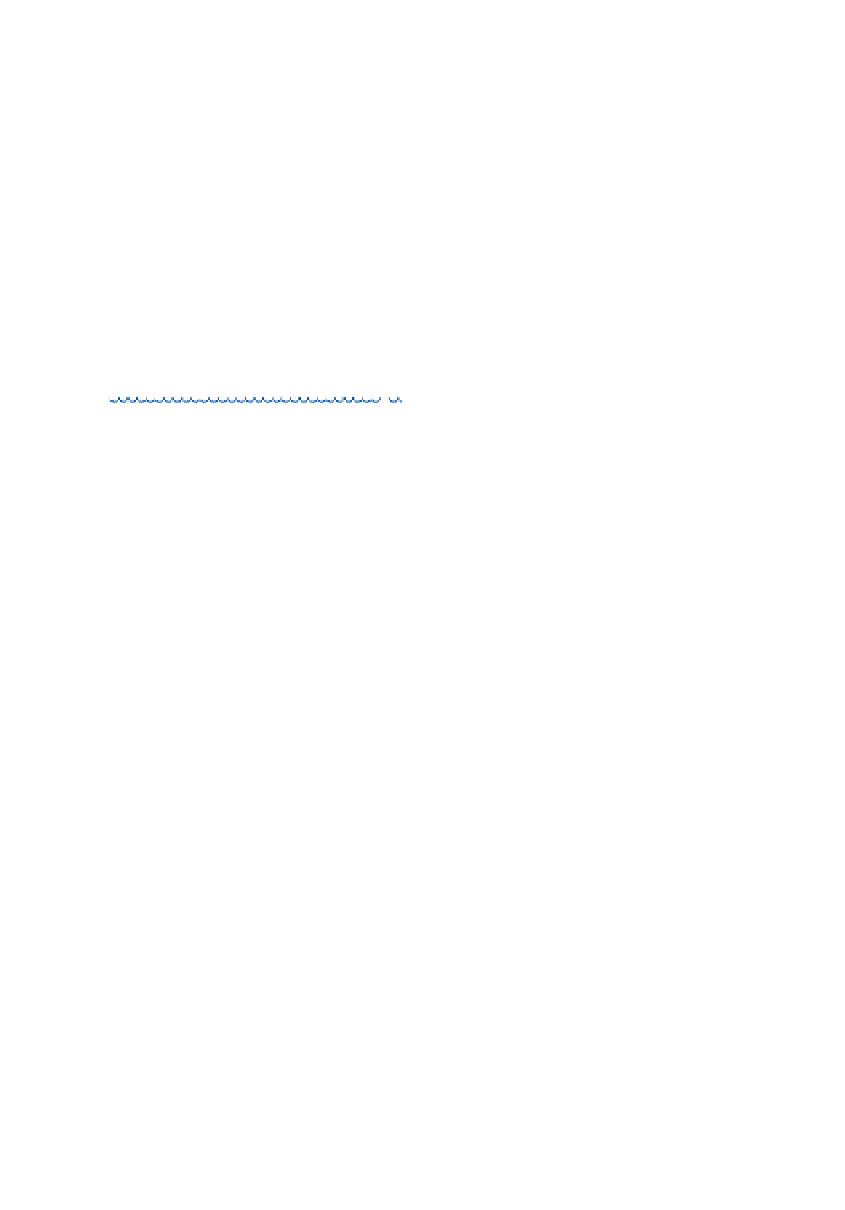







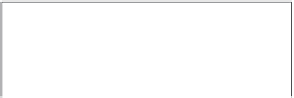
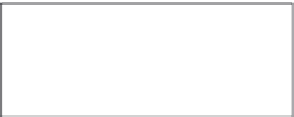
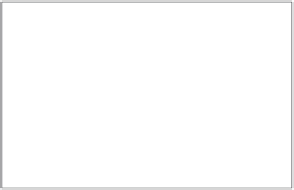











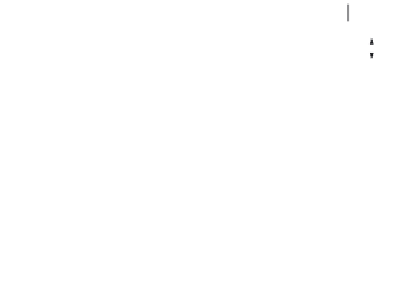
















