Biomedical Engineering Reference
In-Depth Information
p
0
= 0.29 MPa
p
0
= 0.39 MPa
p
0
= 0.57 MPa
80
60
80
60
40
20
0
80
60
40
20
0
40
20
0
-20
-20
-20
0
0.4
Time (
µ
s)
0.8
0
0.4
Time (
µ
s)
0.8
0
0.4
Time (
µ
s)
0.8
FIGURE 6.13
Simulated and measured nonlinear pressure waveforms generated at the focus of a spherical ultrasound transducer evaluated for
different pressure normalization factors. (After M. S. Canney, M. R. Bailey, L. A. Crum, V. A. Khokhlova, and O. A. Sapozhnikov,
J. Acoust. Soc.
Am.
, 124, 4, 2008.)
rectal wall distances and for two ultrasound transducers with
different focal distances, selects the transducer and determines
the power settings, the excitation frequency, and the exposure
time. By combining parameterized 3D anatomical models with
an algorithm that optimizes the lesion locations, patient treat-
ment planning reduces the treatment time for these transrectal
ultrasound applicators with an approach that is readily adapted
to different probe parameters.
Other related numerical models simulate HIFU for ablation
therapy and hemostasis. One simulation model, which is com-
pared to measurements in water and in a gel phantom, computes
the nonlinear pressure fields generated by a spherically focused
HIFU transducer with the KZK equation (Canney et al. 2008).
The simulation results in Figure 6.13 show that as the scale fac-
tor for the input pressure increases, the calculated shock fronts
are increasingly steep with sharper pressure peaks. The simu-
lated pressure waveforms demonstrate close agreement with the
measured waveforms for lower focal intensity values, but some
differences are observed at higher focal intensities. This non-
linear model is ultimately intended for pressure and intensity
calculations in tissue. Another simulation model combines the
KZK equation with a bioheat transfer model and evaluates the
influence of nonlinear ultrasound propagation on transient and
steady state temperatures (Curra et al. 2000). The simulation
results for homogeneous tissue show that nonlinear propagation
strongly influences the peak temperature and the rate of tem-
perature increase in the focal zone and that smaller differences
relative to linear ultrasound propagation models are observed
elsewhere. When a large blood vessel is included in the bioheat
transfer model, the temperature values decrease and the loca-
tion of the peak temperature shifts in the linear and the nonlin-
ear pressure simulations. The large blood vessel, which is located
coaxially with respect to the spherically focused transducer, also
eliminates the enhancement of the temperature deposition due to
nonlinear ultrasound propagation (Figure 6.14).
resonance images, which provides temperature feedback during
a treatment. Some examples of magnetic resonance-compatible
phased arrays constructed for ablation therapy include a
concentric-ring array (Fjield et al. 1996) and a spherical section
array (Daum and Hynynen 1999). hese arrays generate pressure
fields that are numerically modeled with the Rayleigh-Sommerfeld
integral, the resulting temperatures are modeled with the bioheat
transfer equation, and then the thermal dose is computed.
As ultrasound phased array systems with larger apertures
are developed for thermal ablation, the effect of aberrating lay-
ers grows increasingly important, and numerical models that
account for aberrating bone and tissue structures are needed.
One model of curved tissue layers subdivides curved surfaces
into smaller planar radiators, and the contributions from these
planar radiators are superposed (Fan and Hynynen 1994). This
numerical approximation is applied to linear ultrasound propa-
gation through a human skull model obtained from magnetic
resonance images, and the results of phase aberration correc-
tion is evaluated for several spherically focused phased arrays
(Sun and Hynynen 1998). For these simulations, the diameter
and radius of curvature are constants, and the number of ele-
ments within the array aperture is varied. The results show
that refocusing is more effective when more array elements are
defined within the available aperture and that phase aberration
correction is less effective with fewer array elements. Another
simulation model extracts the skull geometry from CT scans
of the head and then computes the pressure in a plane directly
above the skull surface by backward projecting pressures calcu-
lated with the Rayleigh-Sommerfeld integral using the angular
spectrum approach (Clement and Hynynen 2002). The resulting
pressure is then forward propagated through the skull and into
the focal plane for each array element, and the effects of phase
aberration correction are reduced with a refocusing algorithm.
These simulation models also describe the effect of phase aber-
ration on focused ultrasound fields produced during ablation of
uterine fibroids (Liu et al. 2005, White et al. 2008). These models
of ultrasound propagation in the abdomen, which are obtained
from segmented magnetic resonance images, demonstrate that
focal patterns are distorted by the tissue layers and that the dis-
tortion is significantly reduced by phase aberration correction.
6.3.3.4 ablation therapy with
Ultrasound phased arrays
The thermal lesions generated by an ultrasound phased array sys-
tem are often guided by thermometry obtained from magnetic
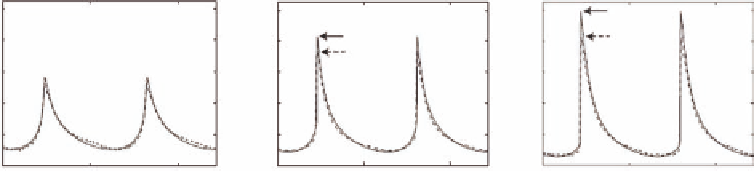