Geoscience Reference
In-Depth Information
radiation outside of the melt season is negligible because of
the small amount of solar radiation reaching the surface and
high surface albedo (Figure 4). Figure 5 from
Intrieri et al.
[2002a] shows annual cycles of the downwelling, upwelling,
and net radiative fluxes and cloud radiative forcing with re-
spect to each flux. During the melt season, from early July
until mid-August, clouds reduce the downward solar flux
reaching the surface by about 100 W m
-2
(Figure 5a). At the
same time, cloud effect on the surface net SW flux strongly
depends on the surface albedo. The cloud SW forcing with
respect to the surface net SW flux is small until the end of
May when sea ice is covered by snow with albedo of about
0.85 (Figure 4). The importance of cloud cooling increases
dramatically with the progression of melt, when the surface
albedo is lowered by snow melting and formation of melt
ponds. Figure 4 shows a large drop in the surface albedo dur-
ing June-July over the sea ice surface without even includ-
ing the melt ponds. While downwelling SW flux reaches 200
W m
-2
in May, the net SW flux is below 50 W m
-2
until the
onset of melt in June when the net SW flux doubles (Figure
4). The largest cloud effect on the surface net SW flux occurs
in the beginning of July (50 W m
-2
, Figure 5a), when surface
albedo is the lowest.
The cloud forcing with respect to both the downwelling
and net LW flux is large throughout the year with the annual
range of 45 W m
-2
(from the minimum of 15 W m
-2
in De-
cember to the maximum of 60 W m
-2
in September) (Figure
5b). During the winter, the downwelling LW flux is the larg-
est source of energy for the surface, and changes in the win-
ter surface air temperature are closely tied to changes in the
LW radiation budget [
Walsh and Chapman
, 1998]. The LW
cloud forcing is dominated by the downwelling component,
while the effect of clouds on the upwelling LW flux is small
[
Intrieri et al.
, 2002a].
Cloud surface longwave forcing depends mostly on the
cloud emissivity (or opacity) and cloud emitting tempera-
ture [
Chen et al.
, 2006]. Because of the frequent presence
of liquid water in the Arctic clouds, their opacity is mostly
determined by the cloud liquid water path (cloud liquid wa-
ter content integrated over the entire atmospheric column).
Cloud longwave forcing is highly sensitive to the cloud LWP
for low LWP values, while the impact of changes in LWP on
cloud longwave forcing is practically zero for LWP values
greater than 30 g m
-2
. This property of clouds is known as
the longwave saturation effect; that is, a cloud with large
LWP emits as a blackbody, and thus any further increase in
its LWP has no effect on the cloud longwave forcing.
The cloud emitting temperature, in turn, is most often
determined by the cloud base temperature. Large values
of the downwelling LW flux correspond mostly to clouds
with base temperatures warmer than -15°C (Figure 6a)
[
Shupe and Intrieri
, 2004]. Some clouds with colder cloud
base temperature are almost indistinguishable from clear-
sky emission. The strongest longwave cloud forcing was
also found for clouds residing at heights lower than 0.5 km
(Figure 6b) [
Shupe and Intrieri
, 2004]. Cloud base height,
however, has mostly an indirect effect on cloud longwave
forcing by influencing the cloud base temperature.
Chen
et al.
[2006] showed that the clouds with bases between 1
and 3 km have decreasing longwave forcing with increas-
ing cloud base height, while clouds with a base height lower
than about 0.6 km have increasing longwave forcing with
increasing height. This relationship shows if the cloud
resides in or above the temperature inversion layer (typi-
cally about 1 km). The cloud base temperature effect on the
LW cloud forcing is enhanced when clouds reside at or near
the peak of the near-surface temperature inversion layer. It
has to be taken into account that when a cloud resides be-
low the temperature inversion layer, the maximum emitting
Figure 4.
Annual cycle of downwelling longwave flux (LW down,
thick grey line), downwelling shortwave flux (SW down, thin black
line), net shortwave flux (SW net, thick black line), and surface
albedo (Alb, dashed line), based on SHEBA observations from 1
November 1997 to 30 September 1998. The daily radiative fluxes
form a composite data set in which 82% of the observations are
from the Atmospheric Surface Flux Group (ASFG) radiometers,
6% are from the Atmospheric Radiation Measurement (ARM) pro-
gram radiometers, 7% are from the SHEBA Project Office (SPO)
radiometers, and 5% are from climatology [
Lindsay
, 2003]. Albedo
data are provided by H. Huwald (personal communication, 2004).
Surface albedo is fixed at 0.85 during winter months. Year day
(1997) zero is defined as 1 January 1997, 0000 LT. Year days for
1 December, 1 March, 1 June, and 1 September are 335, 425, 517,
and 609, correspondingly.










































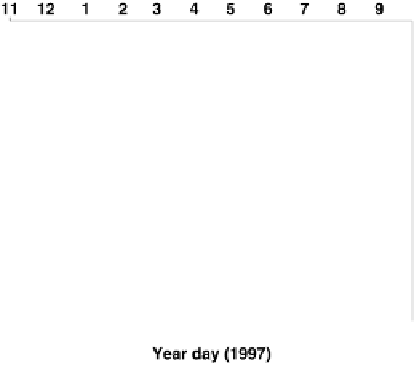








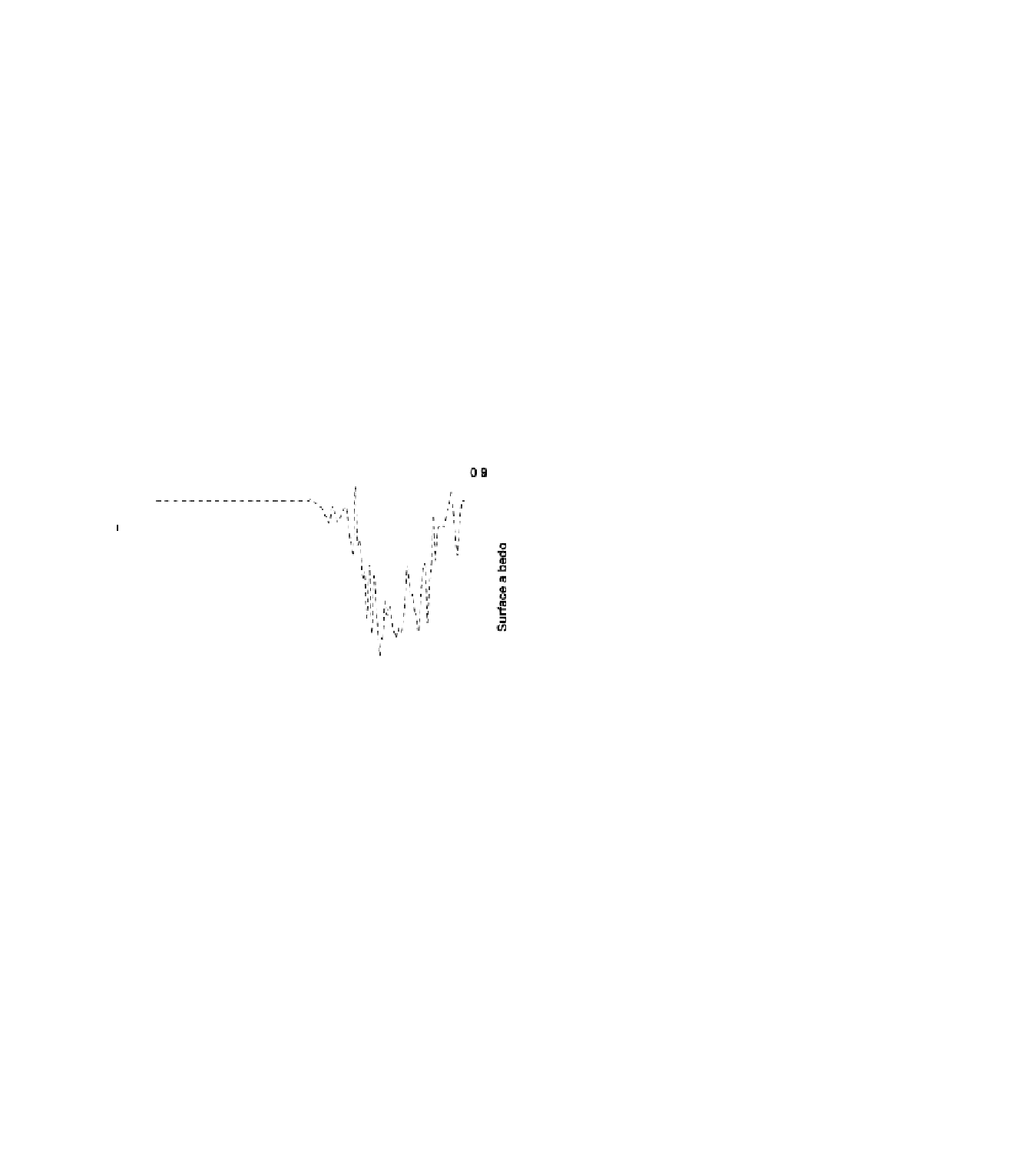























Search WWH ::

Custom Search