Geology Reference
In-Depth Information
South
Windward-Focused Glacial Erosion
North
Apatite He age
Regional A-He
age trend
LGM ELA trend
25
ELA
front
3
max.
elev.
mean
elev.
min.
elev.
20
2
15
rapid
erosion
prevailing winds
10
5
0
1
n = 32
0
20
40
60
120
80
100
Distance (km)
Fig. 10.40
Enhanced glacial erosion on the upwind flank of the St. Elias orogen, southeast Alaska.
Young (U-Th)/He ages in a zone between 35 and 60 km on the upwind flank of the St. Elias orogen imply rapid
erosion that coincides spatially with the position of the glacial-age equilibrium line. The observation that the
deformation front has propagated outward 10-15 km during the past 800 kyr appears inconsistent with the contention
that enhanced glacial erosion caused a contraction of the zone of deformation, as might be expected from theory.
Modified from Berger and Spotila (2008).
eyes are attracted to the 7- to 8-km high peaks,
but only 2-4% of the topography lies above
6 km. Most of the land surface is concentrated
near the snowline. As a result, if rock uplift
drives surface uplift, more of the land surface
is pushed into the accumulation zone for the
glaciers. This enhanced accumulation zone,
too, will enhance the ice flux and, hence, glacial
erosion (Brozovi ´
et al
., 1997). This response is
an interesting example of a negative feedback
in which an increase in mean elevation creates
the conditions that should lead to a lowering of
the mean elevation through enhanced erosion.
In the St. Elias orogen of southern Alaska
(Fig. 10.40), a zone of rapid erosion has been
delineated based on apatite (U-Th)/He cooling
ages (Berger
et al
., 2008; Berger and Spotila,
2008). This zone correlates spatially with the
position of the glacial equilibrium line during the
Last Glacial Maximum and is interpreted as a
response to repetitive snowline lowering during
Middle to Late Pleistocene time. As a consequence,
enhanced glacial erosion has been invoked as
positive feedback that locally accelerates the rate
of rock uplift. Moreover, this region has been
cited as an example of an orogen where a
climatically modulated increase in erosion caused
a reduction in the cross-sectional area of the
orogen (Fig. 10.2) (Berger
et al
., 2008; Whipple,
2009). Such a reduction implies that the leading
edge of deformation retracts during the interval
of enhanced erosion (Davis
et al
., 1983). Although
the leading edge of deformation can be difficult
to define in the past, thickening and thinning of
rather well-dated growth strata in the offshore
seismic stratigraphy from this region (Bruns and
Schwab, 1983; Meigs
et al
., 2008) actually suggest
that thrust sheets have been steadily propagating
outward and the orogenic belt has been widening,
rather than contracting as speculated, during this
Middle to Late Pleistocene interval of rapid glacial
erosion (Meigs, 2010). This apparent contradiction
of a modern paradigm provides a cautionary
note against attempts to generalize both orogen
behavior and climate-tectonic interactions on the
basis of modern snapshots of cooling ages and
snowlines in the absence of careful attention
to spatial and temporal reconstructions of the
structural history of an orogen.
One persistent, but unresolved, problem
relates to the rate at which glaciers can actually
erode bedrock. Is it as fast as or faster than riv-
ers? The fact that moving ice covers the bed of
glaciers makes it difficult to observe and cali-
brate erosion processes at the glacial bed. A
qualitative answer, however, can be derived sim-
ply by examining the topography of glaciated
and non-glaciated regions. At least two observa-
tions suggest that glaciers erode bedrock at least
as fast as rivers do. First, when side-by-side










































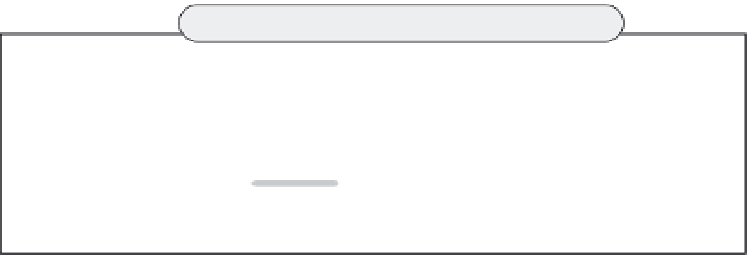














































