Geology Reference
In-Depth Information
for stationary dislocations between two subsequent etch-
ing and flat bottomed pits for the defects that moved
some time after the first application of the etchant. In
case of ice, there is an added advantage due to the high
temperature because of the higher thermally activated
mobility. If the dislocations move during etching, etched
tracks can then develop. The tracks were used as evi-
dence for the mobility of these defects. However, basal
dislocations believed to be responsible primarily for
creep, deformation, and general viscoelastic flow of ice
could not be identified by etch pitting and were unani-
mously considered by the ice researchers to be indistinct
until 1977.
Glide dislocations in the basal plane are considered to
take the dominating role in the strength properties of ice.
These dislocations emerge on the prism planes of the
crystal. They have been observed by X‐ray techniques
[
Fukuda and Higashi,
1973;
Jones and Gilra,
1973] in care-
fully prepared single crystals of ice of low dislocation
density. The X‐ray diffraction techniques cannot be
applied to ordinary polycrystalline ice because of the
limitations imposed by the resolution of the method and
other inherent difficulties, particularly due to overlapping
shadow effects. It was realized that the etch pitting tech-
nique requires further development in order to be used to
reveal glide dislocations in the basal plane [
Sinha,
1977b].
The preferential removal of surface molecules from a
crystal to the surrounding medium (air or liquid) is the
basic factor that controls the etching process. This prefer-
ential dissolution is governed by the difference in free
energy between the undisturbed surface and the site of an
imperfection. The surface free energy, as a measure of the
difference between the energy of an atom at the surface
and one in the interior, is a function of temperature and
of the medium to which the surface is exposed. In case of
ice, the molecules are water and they are at extremely high
energy states due to homologous temperatures close to its
melting point.
The phenomenon of preferred dissolution of atoms at
dislocation emergence sites in solids have been analyzed
theoretically by
Cabrera
[1956]. He came to the conclu-
sion that the success of forming good quality etch pits
at the sites of imperfections would depend on how much
the surface energy was decreased near the defects by the
imposed environmental conditions of etching. The analy-
sis bears close resemblance to the description of crystal
growth and prefers evaporation (sublimation) by a dislo-
cation mechanism given by
Frank
[1952]. This description
appears to be reasonable for the case of thermal etching
of ice under saturated conditions as can be appreciated
from a study by
Krausz and Gold
[1967].
The difference in free energy between the point of
emergence of individual dislocation lines and the surface
is small in comparison with differences associated with
(a)
(b)
γ
VS
γ
VS1
γ
VS2
γ
VS
θ
vs
θ
VS
Grain 1
Grain 2
Grain 1
Grain 2
γ
gb
γ
gb
Figure 6.25
Thermal etching of (a) asymmetrical grain
boundary and (b) symmetrical grain boundary (N. K. Sinha,
unpublished).
grain boundaries. The latter are well-developed slip planes
and relatively larger small-angle boundaries containing
numerous dislocations [
Weertman and Weertman,
1964].
Saturating the environment surrounding the ice specimen
appears to have controlled the surface energy to a suffi-
cient degree as to allow
Krausz and Gold
[1967] to observe
thermally etched features corresponding to large linear
imperfections at −10 °C but not dislocations. Observations
made by the co‐author of this topic (N. Sinha) at −30 °C
suggested that dislocation bundles and grown‐in disloca-
tions could be readily revealed by this method. It was
apparent that the thermal etching of ice in air depends on
both relative humidity and temperature, and that there
were potentials of this method yet to be established.
Grain boundary grooves for any polycrystalline or bi‐
crystalline develop at the vapor‐solid or liquid‐solid
interface (Figure 6.25). The grooves depend on the “spe-
cific surface free energy” or simply “surface energy”
defined as the amount of work necessary to create a unit
area of the interface. Formations of the grooves allow the
detection of the grain boundaries and often subgrain
boundaries (especially for sea ice to be shown later). If
the vapor‐solid grain boundary groove angle between
grain 1 and grain 2 is
θ
VS
(sum of the two angles
θ
VS1
and
θ
VS2
for the two grains with respect to the grain boundary
inclination), then a simple relationship can be given for
equilibrium condition:
/
1
/ cos
cos
(6.3)
VS
1
VS
2
gb
VS
1
VS
2
where
γ
gb
is the grain boundary energy and
γ
VS1
and
γ
VS2
are the surface energies of the exposed crystalline faces of
the two grains with respect to vapor. If it is assumed that
the surface energy does not depend on the crystalline sur-
face exposed to the medium; then for a symmetrical
boundary, the relationship can be simplified to
/
12
cos
/
2
(6.4)
VS
gb
VS
















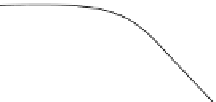






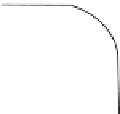





