Chemistry Reference
In-Depth Information
(a)
(b)
O
O
OH
O
O
O
OCH
3
N
P
Fluorescence OFF
N
O
PPh
2
N
N
N
N
O
O
O
Quencher
R-N
3
/water
NO
2
O
O
OH
R
O
NH
O
O
P
O
R
N
H
Fluorescence ON
N
O
O
N
PPh
2
O
fIgure 2.2
Fluorescence switch upon Staudinger ligation. Fluorescent was quenched by an electron lone pair from phosphorus (a) and
a quencher (b).
utilising bertozzi-Staudinger ligation have been reviewed extensively elsewhere [53, 54]. Here, we will focus on the appli-
cation of this reaction in the introduction of molecular imaging probes.
using the original Staudinger ligation approach, phosphine-conjugated fluorescent labels, Cy5.5, fluorescein, and rhoda-
mine, were introduced to living cells by visualised cell surface glycans after metabolic labelling with azido groups [55].
besides the direct fluorescent labelling of the azide-modified biomolecules, the bertozzi group also developed 'switch-on'
probes upon Staudinger ligation. one of the probes was a coumarin-phosphine conjugate, where the fluorescence of coumarin
was quenched by the electron lone pair of the phosphine phosphorus (Figure 2.2
a
) [56]. upon reaction with azido-protein,
coumarin was attached to the protein and fluorescence was turned on. Another example employed a dye-quencher system,
wherein a fluorescein analogue and disperse Red 1 were attached to the same phosphine molecule (Figure 2.2
b
) [57]. once
Staudinger ligation occurred on the azidoglycan-expressing cell surface and was obtained after metabolic labelling, the
quencher molecule disperse Red 1 was cleaved off, turning fluorescence on.
bioluminescence imaging of these engineered cell surface azido-glycans was evaluated by feeding phosphine-linked luciferin
to luciferase-expressing cell lines [58]. Staudinger ligation released the free luciferin, which diffused into cells, where it served
as a luciferase substrate leading to light emission. Although only visualisation of engineered cell surface glycan was reported,
these strategies may find their way in the imaging of other azide-modified molecules in cells and whole animals.
despite the widespread use of Staudinger ligation
in vitro
and in living cells, only a handful of reports are available for its
application in medical imaging
in vivo
. Following bertozzi's initial nontraceless Staudinger ligation work, a recent report applied
intravenous injection of neutrAvidin, labelled with either a far-red fluorophore dyLight649 or doTA-
111
In, after the same met-
abolic engineering and Staudinger ligation as that in bertozzi's work [59]. The researchers found significant azido-labelled
N
-acetyl-mannosamine-dependent increase in tissue contrast, which was detected using optical imaging and single-photon-
emission computed tomography (SPeCT). Although this case showed great promise for Staudinger ligation in nuclear imaging,
another group could not reproduce the successful use of this reaction in live animals [60]. In this study, researchers attempted to
introduce radiolabels to tumours in a two-step approach, first pretargeting the tumour with azido-antibody followed by radiola-
belling of antibody with phosphine derivatives via Staudinger ligation. The advantage of the approach is obvious, because it
could reduce the radiation of a directly radiolabelled antibody, which has longer circulation half-life than radiolabelled phosphine
probes, such as
89
Zr- and
67/68
ga-labelled desferrioxamine-phosphines,
177
Lu-doTA-phosphine and
123
I-cubyl phosphine probes.
However,
in vitro
Staudinger ligation between the azido-antibody and phosphine probes was not efficient in the presence of
serum, and a side product of phosphine was identified; no
in vivo
Staudinger ligation product was observed in the mouse model.
Clearly, extension of the reaction in living system would require further investigation and optimisation.
2.2.2.2 Azide-Alkyne Cycloaddition
Huisgen 1,3-dipolar cycloadditions are exergonic reactions to condense two unsat-
urated molecules to yield various five-membered heterocycles [14]. Among them, azide-alkyne cycloaddition is arguably
the mostly explored and used reaction; it was originally performed at elevated temperatures, giving a mixture of 1,4- and
1,5-cycloaddition isomers. The Sharpless group and the Meldal group independently discovered that copper (I) could greatly
accelerate the reaction rate (by seven orders of magnitude compared with reactions without copper) at lowered temperatures
leading to products with higher regioselectivity (1,4-isomer only) and often nearly quantitative yields (Scheme 2.5) [61, 62],
making the azide-alkyne cycloaddition one of the most popularly used conjugation methods.
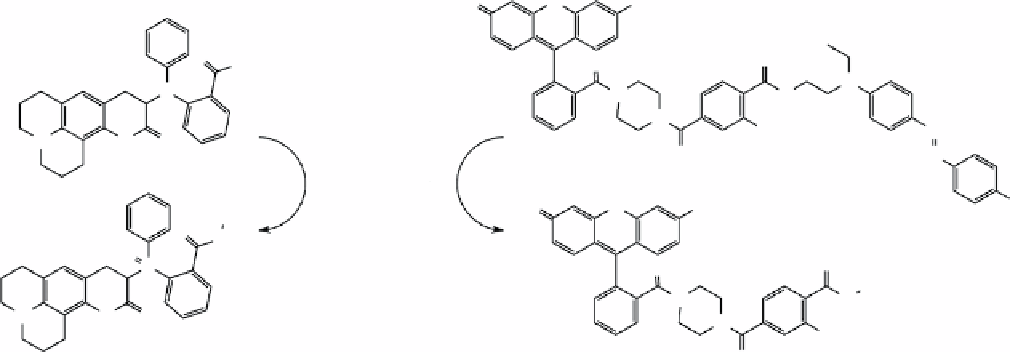