Chemistry Reference
In-Depth Information
100
80
2-
O
3
P
PO
3
2-
NN
NN
60
Tm
2-
O
3
P
PO
3
2-
40
[Tm-DOTP]
4-
20
0
60
40
20 0
Saturation frequency-ppm
-20 40
-60
fIgurE 10.13
CEST spectra of a solution of 0.11 mM poly-l-arginine alone (open squares) and 0.11 mM poly-l-arginine in the
presence of 2 mM of Tm(HDOTP)
4-
(filled squares) acquired at 7.05 T, pH 7.4, and 312 K. Irradiation conditions: square pulse, duration:
2s, power: 25.0 μT. Reproduced with permission from Ref. [8].
very likely that the Tm(HDOTP)
4-
chelate sweeps along the polypeptide surface, thus causing an overall shift of all guanidine
protons that, upon saturation, resulted in a strong decrease in the water signal intensity. An 18:1 ratio between the paramag-
netic chelate and the polypeptide was found to provide an optimal CEST effect, whereas higher ratios yielded extensive
precipitation of the adduct owing to the decrease of the effective residual electric charge.
Interestingly, a 5% CEST effect was observed at very low concentrations of polymer (1.7 μM) and metal complex (30 μM),
thus demonstrating the great sensitivity enhancement that can be attained by this approach. Although the observed sensitivity
enhancement significantly decreased the detection limit of an MRI-CEST experiment to values that approached conven-
tional Gd(III)-based contrast agents, a further step toward high sensitivity CEST probes has been attained by the introduction
of lipoCEST agents.
10.7
lipocESt agEntS
A dramatic increase in sensitivity has been obtained by considering the ensemble of water molecules contained in the inner
cavity of liposomes as a pool of exchangeable protons. Depending on the size of these liposomes (50-300 nm in diameter),
the number of exchangeable protons can be as high as 10
6
to 10
8
per liposome. In order to distinguish between the internal
and external water protons, a paramagnetic shift reagent (SR) must be entrapped in the inner aqueous cavity. ln(III)DOTA-
like complexes, in which the ninth coordination site around the lanthanide ion is occupied by a water molecule in fast
exchange with the bulk molecules, have been shown to work very well in this respect. The coordinated water molecule lies
along the molecular axis, and this structural arrangement endows the water protons with a large hyperfine shift. In Figure 10.14,
the
1
H-NMR spectrum of such a suspension containing a lipoCEST agent (Tm-DOTMA as SR) is shown [69, 70].
lipoCEST agents display exceptional sensitivity in the picomolar concentration range (on a per liposome basis) without
the need for high saturation powers. However, the major disadvantage of first-generation lipoCEST agents lies in the small
chemical shift difference between the inner water resonance (±4 ppm depending on the Bleaney constant of the lanthanide
ion of choice) and the bulk water signal. Hence, the
in vivo
application of these lipoCEST agents is limited by the fact that
endogenous molecules may exhibit exchangeable protons in the same frequency region. To overcome this drawback, it is
necessary to develop lipoCEST agents with much larger separation between the inner water and the bulk water resonances
(Δ
int
ralipo
), a feature that can be achieved by either changing the nature and concentration of the SR unit or by altering the
shape of the liposome. Basically, Δ
int
ralipo
is the sum of two contributions: (i) the chemical interaction arising from the labile
coordination of a water molecule to the metal centre of the SR (pseudocontact shift, Δ
pseudo
), and (ii) the changes in bulk
magnetic susceptibility (BMS shift, Δ
BMS
) due to compartmentalisation of the paramagnetic SR inside the vesicle (Eq. 10.2).
int
ralipo
pseudo
BMS
∆
=
∆
+
∆
(10.2)
The latter term is null for spherical compartments but can dominate for asymmetric systems (Figure 10.15) [71]. It is
worth noting that both the ∆
pseudo
and ∆
BMS
contributions are directly dependent on the intraliposomal concentration of the SR,
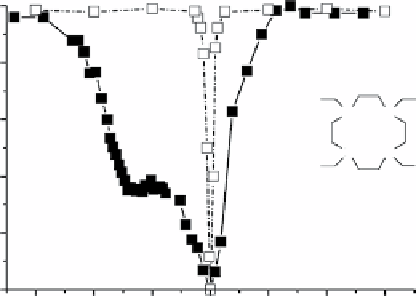