Chemistry Reference
In-Depth Information
images after a normal intravenous injection of a Eu
3+
-based PARACEST agent [57]. The T
2exch
effect is obviously detrimental
to CEST imaging because the signal loss is present even before a frequency-selective RF pulse can be applied to activate the
PARACEST agent. Thus, the desired CEST signal contributed by the sensor is masked by the less desirable T
2exch
contribu-
tion to the image. Although the above effect has slowed translation of these water exchange-based PARACEST agents into
animal imaging, this new mechanism could pave the way for a new class of activatable T
2exch
agents.
Nevertheless, there have been a few reports of successful detection of PARACEST agents
in vivo
and in isolated, perfused
tissues (
ex vivo
). The first report demonstrated the use of a train of 360°WAlTZ-16* preparation pulses applied directly on
bulk water (the OPARACHEE method) to provide a direct measure of water signal loss caused by the paramagnetic agent.
This method essentially presents an indirect way to detect the agent without the need of applying a frequency-selective pre-
saturation pulse to activate the agent. Given that a Tm
3+
-based PARACEST agent was found to be optimal for OPARACHEE
and that this Tm
3+
-based agent also induces a much larger hyperfine shift in its bound water molecule compared to the more
common Eu
3+
-based agents, this effort effectively represented the first report of the detection of a very efficient agent that
can now be referred to as a T
2exch
agent. Nonetheless, it was the first imaging method used to successfully monitor the
accumulation and clearance of a PARACEST agent in mouse kidney [58]. More recently, OPARACHEE was also success-
fully used to detect a different PARACEST agent in mouse kidney [59] and in a mouse glioblastoma multiforme tumour
[60]. Additionally, the contrast from PARACEST agents conjugated to second-generation and fifth-generation polyamido-
amine (PAMAM) dendrimers has also been used to track the relative pharmacokinetics of these nanoparticles
in vivo
[61].
Following the injection of the dendrimer conjugates into a mouse model of mammary carcinoma, a gradual increase in image
contrast resulting from accumulation of the PARACEST conjugates in the tumour was observed.
The same Eu
3+
-based glucose sensor described in Figure 10.9 has been used to image the tissue distribution of glucose in
perfused mouse livers [62]. This was accomplished by perfusing two different livers to steady state, one with the agent plus
10 mM glucose and the second liver with the agent alone. Comparisons were then made of the water image intensities in the
two livers upon activation of the PARACEST agent by a long pre-saturation pulse at 42 ppm (the chemical shift of the Eu
3+
-
bound water exchange peak). Interestingly, the liver perfused with the agent plus glucose showed a 17% decrease in water
intensity compared to the liver with the same amount of agent but no glucose (Figure 10.11). This important experiment
(a)
100
80
Δ
Glc
60
40
37°C
No Glc
1:1 Glc
20
ω
on
120
90
60
Saturation offset (ppm)
30
0
-30
-60
(b)
(c)
(d)
Δ
Glc
30%
Glc free
10 mM Glc
0%
Off
On
CEST
fIgurE 10.11
(a) CEST spectra of fresh effluent from a liver isolated from a 24-hr fasted mouse and perfused at 37°C showing a glucose-
induced CEST peak at 42 ppm. Both perfusates contained 10 mM agent at pH = 7.4. (b) single slice selected images of mouse livers col-
lected without a pre-saturation pulse (off') and (c) with a pre-saturation pulse (on). The two livers were perfused using a physiological
buffer without glucose (top) or with 10 mM glucose (bottom). Both perfusates contained 10 mM agent at 37°C. (d) The difference CEST
image showing the glucose induced CEST contrast between the fed and fasted mouse livers. Reproduced with permission from Ref. [62].
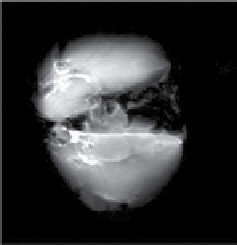
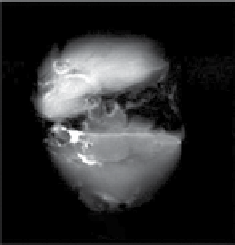
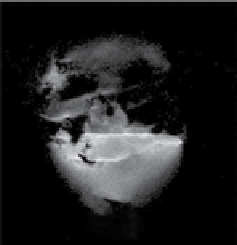
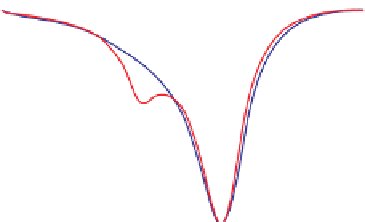


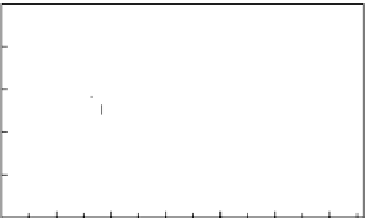





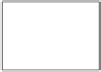






