Chemistry Reference
In-Depth Information
(a)
(b)
(c)
(d)
(e)
4%
8
-6%
5
fIgurE 10.2
APT effects during focal ischemia in rat brain. No effect of ischemia was visible on the T
2
T2-weighted image (a), but the
pH-sensitive images (d and e) show the ischemic region. As confirmed by the diffusion weighted image (b), and histology (c). Adapted
with permission from Ref. [18].
results in an interesting phenomenon of generating little to no CEST contrast at low pH, maximal CEST at pH values
between 6 and 8 depending on the compound, then a loss in CEST signal again at high pH values [19]. Given that the mag-
nitude of CEST contrast is heavily influenced by
k
ex
, the CEST signal can be used to monitor changes in tissue pH. This
strong pH dependence of the APT signal has been used to detect ischemic regions of rat brain tissue [18, 20]. In this case,
ischemic tissue resulting from lack of sufficient oxygen delivery also results in a decrease in tissue pH and a corresponding
reduction in APT contrast (Figure 10.2). APT could prove useful for detecting early ischemic events such as those occurring
in stroke where conventional MRI methods are not particularly sensitive.
APT imaging has also been used to detect brain tumours in both humans and animals by taking advantage of their higher
protein/peptide content compared to normal brain tissue. When applied to rats implanted with 9 l gliosarcoma tumours, the
APT signal outlined a well-defined brain tumour region and was able to distinguish between edema and tumour, whereas T
1
,
T
2
, and diffusion-weighted MRI methods yielded a more diffuse tumour pattern [17]. The above technique was extended into
imaging human brain tumour, and the results obtained were very consistent with the animal studies [21]. More recently, the
potential of utilising APT imaging for tumour grading and tumour zone delineation was noninvasively demonstrated in
human brain tumours [22, 23]. APT intensities were found to be significantly higher in the core of high-grade tumours than
in normal-appearing white matter (NAWM) and peri-tumoural edema in patients with high-grade tumours, whereas no such
signal differences were observed in low-grade tumours. The utility of APT MRI in the differentiation of viable glioma from
radiation necrosis in rats has also been reported [24].
Exogenous agents with exchangeable amide protons can also be used for CEST imaging. Iopamidol, a clinically approved
X-ray contrast agent having two non-equivalent amide protons that display different exchange rates, has been exploited for
pH mapping
in vivo
[25]. upon injection into healthy mice, the distribution of Iopamidol in the kidney was imaged using
CEST methods, and the corresponding pH map of the kidneys was obtained using ratiometric imaging methods (Figure 10.3).
This study further reinforced the advantage of CEST over conventional MRI methods in providing functional information.
The -OH protons of endogenous molecules have also been used for CEST contrast in tissue. Although -OH protons are
characterised by small chemical shifts and fast exchange, the CEST signal due to the exchange of these protons becomes
more defined at higher magnetic field strengths due to an increase in ∆ω that favours slow-to-intermediate exchange. For
example, glycogen, the primary storage form of glucose in mammalian tissue, constitutes a large number of exchangeable
-OH protons that can be saturated simultaneously by use of a frequency-selective RF pulse. Given the high abundance of
glycogen in liver, the possibility of monitoring glycogen metabolism by CEST in perfused mouse livers at 4.7 T was ele-
gantly demonstrated by van Zijl et al. [26]. upon addition of glucagon to induce glycogenolysis, a reduction in the CEST
signal resulting from glycogen -OH exchange was observed. The reduction in CEST contrast correlated nicely with deple-
tion of glycogen and export of glucose into the medium (Figure 10.4). Because glycogen content may be abnormal in
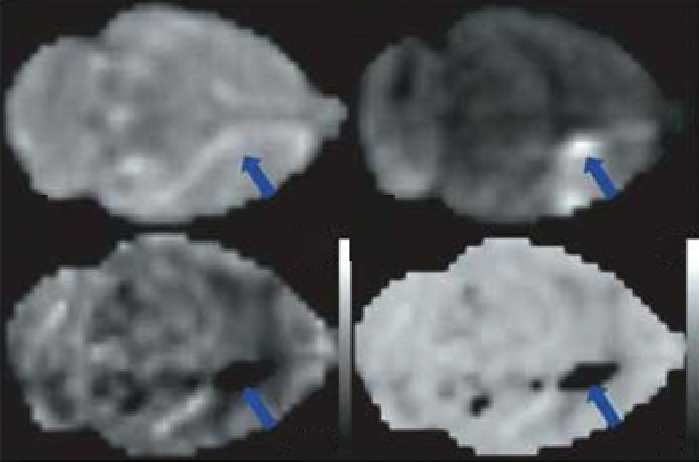
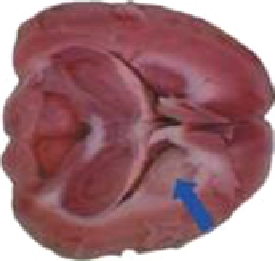