Chemistry Reference
In-Depth Information
H
H
H
H
k
on
N
N
N
N
+
N
N
k
off
N
N
H
H
H
H
L
M
ML
[ML]
[M] [L]
K
ML
=
= Relevant
thermodynamic
stability constant
k
off
=Key kinetic
parameter in vivo
fIgure 5.2
Cartoon representation of basic thermodynamic and kinetic properties of metal-chelate complexes, with k
off
being the most
relevant measure for kinetic inertness
in vivo
.
This is a very important factor because the rate of dissociation
in vivo
is what governs the kinetic inertness of a radiometal
complex and therefore the ultimate suitability of a metal-chelate complex for medical applications. Another important ther-
modynamic parameter, perhaps even more important than
K
Ml
values, is the pM value (-log[M]
Free
) [37, 38]. The pM value
is the negative log of the concentration of free metal ion left uncomplexed by a given chelator. It is calculated under a specific
set of conditions. The pM value takes into account several factors that are not included in the
K
Ml
value, such as ligand
basicity, metal ion hydrolysis, and various other parameters, but it is condition-dependent. When a radiopharmaceutical is
introduced into biological circulation, the extreme dilution may dramatically increase the rate of dissociation, despite a very
high thermodynamic stability (
K
Ml
, pM). One common method employed to access this rate information is acid dissociation
experiments, typically performed in hCl/dCl solutions (often 5-6 M) and monitored via ultraviolet-visible spectroscopy
(uV-Vis) or by nuclear magnetic resonance (nMR) in acidified d
2
O over extended periods of time [35, 36]. A comprehen-
sive table of
K
Ml
and pM values has not been included, because it has been extensively discussed elsewhere [18, 37, 38].
Although
K
Ml
and pM values are very useful,
in vivo
behaviour is ultimately not well predicted using these parameters.
Macrocyclic chelators are generally more kinetically inert than acyclic chelators, even when their thermodynamic stabil-
ities have been determined to be very similar [39-43]. Macrocyclic chelators possess constrained geometries and partially
pre-organised metal ion binding sites, which decrease the entropic penalty paid on metal ion coordination. When a metal ion
is bound, it forces a rigid and constrained geometry on an otherwise freely moving acyclic chelator, so there is a significant
decrease in entropy. The already constrained and pre-organised structures of macrocycles result in a less significant decrease
in entropy on binding, therefore adding a powerful thermodynamic driving force toward complex formation. This phenomenon
is referred to as the 'macrocycle effect,' and is an energetic incentive that macrocycles have over acyclic chelators, which is
in addition to the chelate effect from which they both benefit [44]. Acyclic chelators, however, have much faster coordination
kinetics than do macrocycles, and when properly designed are often able to quantitatively coordinate a radiometal in ~10 min-
utes at room temperature, whereas macrocycles often require heating above 80 °C and longer reaction times (30-90 minutes)
[45-47]. Fast room temperature labelling becomes a crucial property when working with heat-sensitive molecules such as
antibodies and their derivatives, or when working with short half-life isotopes such as
68
Ga and
62
Cu.
Without adequate complex stability, radioactive metal ions may be absconded by blood serum proteins such as transferrin
or serum albumin, decreasing selectivity and contrast from the intended agent, and in extreme cases rendering it useless.
Without adequate radiopharmaceutical uptake, target specificity, and stability, background activity in non-target tissue will
be high and will complicate the collection and interpretation of imaging data, such as delineation of cancerous lesions. Many
different
in vitro
stability assays can be performed to estimate
in vivo
stability; however, the ultimate determinant for the
usefulness of metal-chelate complexes in biological systems is obtained from
in vivo
analysis by biodistribution and/or
imaging studies. Each metal ion will have its own unique biological fate when freed from a radiopharmaceutical
in vivo
,
where they are typically distributed and managed by transport proteins and other biological systems (Figure 5.3).
Translation from test tubes into living organisms presents a multitude of complications. The human body contains many
endogenous ligands (naturally produced chelators/transport proteins/enzymes, etc.) that play vital roles in the metabolism
and homeostasis of metal ions. When evaluating a new radiopharmaceutical agent
in vivo
, the stability can be monitored by
watching for abnormal accumulation of the free nuclide in various organs (where each radiometal ion has been previously
determined to accumulate). Biodistribution studies of radiometal-chelate complexes can be used to evaluate nonspecific
organ uptake (bone, kidneys, liver, lungs etc.) and ensure that the radiometal remains chelator bound and is excreted intact
(typically through the urine and/or faeces). Another negative consequence of radiometal loss
in vivo
is target receptor satu-
ration (Figure 5.3). The concentration of cell-bound receptors in tumours is low, and if there is a significant concentration of
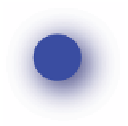
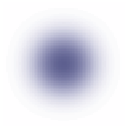
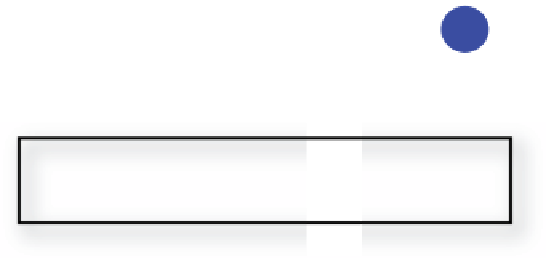
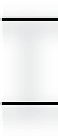























