Geoscience Reference
In-Depth Information
makes it popular, and, despite its shortcomings and its
inevitable inaccuracies, it is one of the more widely used
methods of assessing PE.
In the United Kingdom the Penman formula has also
been widely used for calculating PE. It is less empirically
based than the Thornthwaite method, combining the
energy budget and the aerodynamic approaches to
the estimation of evaporation, but requires much more
meteorological data. Different versions of the basic
formula have been developed. They are based on the
duration of sunshine, or net radiation if measured, mean
air temperature, mean air humidity and mean wind speed.
The data are needed at only one level above the ground
surface and are recorded at many meteorological stations.
The Penman approach has been modified by Monteith
to allow for the nature of vegetation with either optimal
or restricted water supply. Although likely to be more
realistic, the formula does require information about the
resistance of the plants' stomata to water vapour flow. This
is not readily available, so the use of the formula has been
restricted to research applications.
Evapotranspiration in the hydrological
cycle
As we have noted on p. 81, evapotranspiration provides
the main output of moisture from the surface hydrological
system, returning water to the atmosphere. So far we
have discussed some of the processes involved, but it is
important to appreciate that evapotranspiration occurs in
many different stages of the hydrological cycle. Thus losses
of water to the atmosphere may take place at any point
within the system (
Figure 5.1
).
One of the major losses, for example, occurs during
precipitation. Considerable amounts of moisture may be
evaporated during rainfall and the small droplets in
particular may be totally evaporated before they reach
the ground. Similarly, moisture which is intercepted by
the vegetation is also susceptible to direct return to the
atmosphere by evaporation. The amount of moisture
retained on the vegetation during a storm varies according
to the character of the storm, the species of plant, the leaf
density (and therefore the time of year) and, of course,
the vegetation density. In the case of woodland as much
as 50 per cent of the incoming water may be retained in
the canopy and returned as evaporation. In the case of
more low-lying vegetation, such as grass, the amount of
interception is not known with such certainty, partly
because of the difficulty of measuring interception in
crops. Nevertheless, again it seems likely that interception
may reach 20-30 per cent.
A proportion of the water that reaches the soil is also
returned by evaporation, for in heavy storms rainfall often
collects in surface depressions and these are gradually
dried by the sun. Similarly, some of the rainfall flows
across the surface as run-off, and further evaporation
losses may occur at this stage. Rates are generally low,
however, for turbulence mixes the water and disperses the
heat from solar radiation through the water body. Thus
much greater inputs of energy are needed to heat the water
and less is available to carry out evaporation. Nevertheless,
losses at this stage are often important to humanity. Open
canals and reservoirs may lose considerable quantities of
especially, this may represent an irretrievable loss of an
important resource.
In vegetated areas the major process of moisture return
is by transpiration. Rates of transpiration vary according
to the character of the vegetation and therefore change
over both space and time. They are at a maximum when
the vegetation is in full leaf and the soil is moist; they
decline as the plants lose their leaves or the soil dries out.
During the course of a single year, therefore, transpira-
tion losses may show complex fluctuations in response
Moisture budget methods
An alternative method of obtaining actual evapotranspi-
ration is the moisture balance equation. At a site the
moisture balance can be expressed as:
P
+
I
=
E
+
R
+
D
+
S
where
P
is precipitation,
I
is irrigation water added,
E
is
evapotranspiration,
R
is run-off,
D
is drainage to bedrock
and
S
is the change in soil moisture content. As before,
if we knew all the other elements in this equation we could
calculate
E
by difference. Several of the components
present little problem, for precipitation and irrigation
inputs can easily be measured, as can run-off. But drainage
to bedrock (
D
) and changes in soil moisture content (
S
)
are rarely known. As a result, the method can be used only
on a large-scale, long-term (e.g. annual) basis where it can
be assumed that drainage to bedrock is balanced by release
from spring seepage, and where changes in soil moisture
content are negligible.
It is clear from what we have said that evapo-
transpiration remains one of the most difficult aspects
of the hydrological system to measure. For this reason, if
for no other, our knowledge of the processes involved
remains uncertain. For the same reason, it is difficult to
give precise figures for the global pattern of evapotran-
spiration. None the less, it is useful to consider the role of
evapotranspiration within the global system.
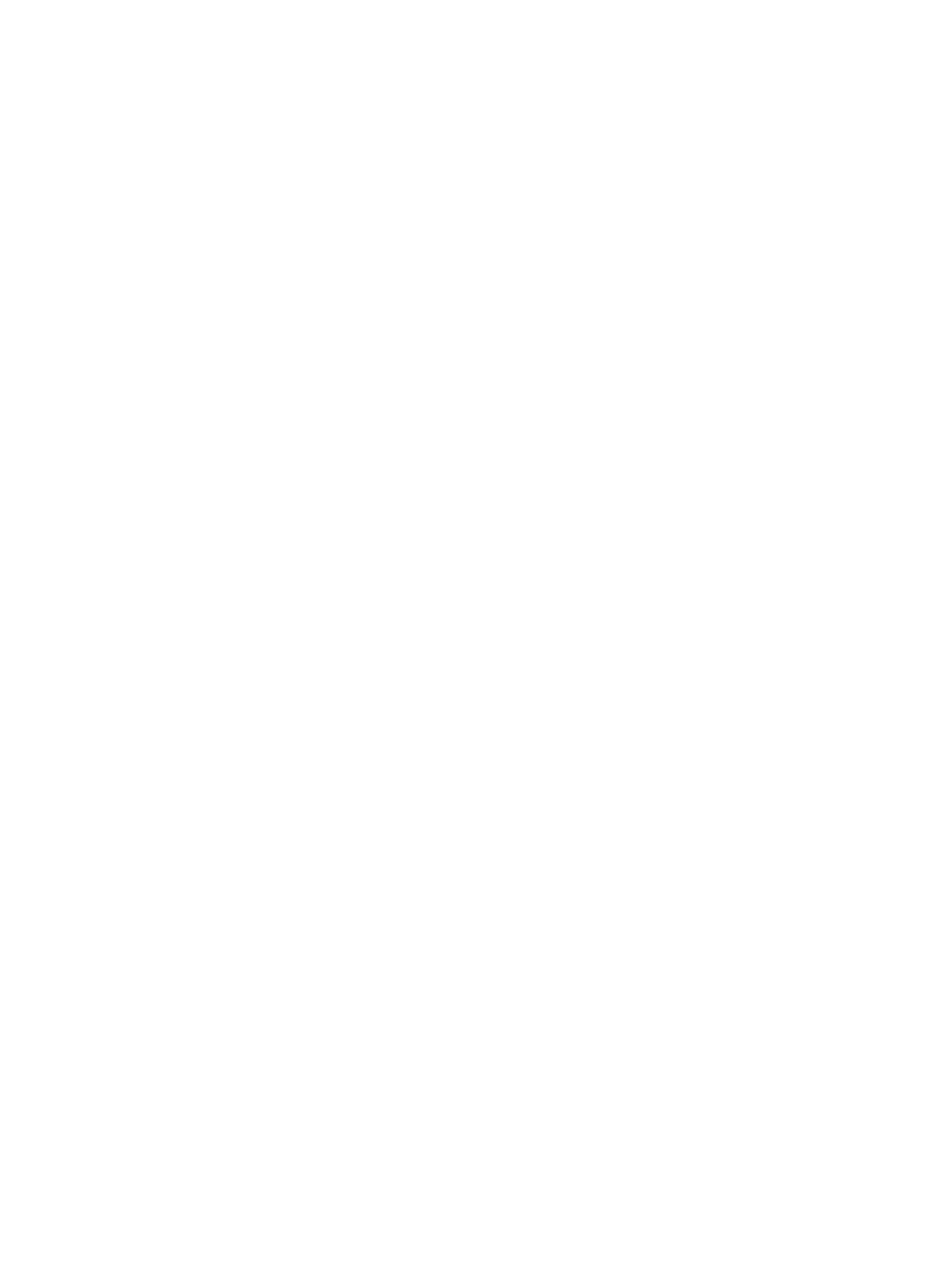






































