Geoscience Reference
In-Depth Information
130
120
110
100
90
80
2
2.5
0
2.5
10
3
10
4
10
5
(a)
130
120
110
100
90
80
22.5
0
2.5
10
3
Electron density
(cm
23
)
10
4
10
5
Wave electric field
(mV/m)
(b)
Figure 4.27
(a) Electron density data from a swept Langmuir probe together with high-
frequency (34-1000Hz) electric field data for a daytime equatorial rocket flight. (b) Elec-
tron density data from a fixed-bias Langmuir probe on a nighttime flight along with
high-frequency (60-1000Hz) electric field data. The plot in (b) corresponds to an off-
equator site (Kwajalein) with a dip angle of 7
◦
. [After Pfaff et al. (1982). Reproduced
with permission of the American Geophysical Union.]
show fluctuations on the downward gradient. Curiously, there are no irreg-
ularities on two of the three layers, even though a dc electric field would be
present at each of them due to the mapping effect. In Chapter 6 we return to
this problem and offer an explanation that does not involve the electric field but
rather the neutral wind as a source of instability. To summarize, the waves are
clearly electrostatic (
n
fluctuations exist), are organized by the direc-
tion of the gradient, and have broadband electric field fluctuations of the order
of several millivolts per meter, which is comparable to the vertical polarization
field.
We turn now to results of the CONDOR daytime rocket launch carried out
on March 12, 1983, from Peru (Kudeki et al., 1987; Pfaff et al., 1987a, b).
The rocket apogee was kept low to maximize the time and horizontal distance
in the electrojet. The zero-order density profile is presented on the left side of
Fig. 4.28 for the daytime electrojet flight, during which the magnetic deflection at
Huancayo was 130 nT. A sonogram of the signals from a plasma wave receiver
δ
E
and
δ
n
/
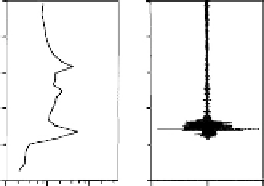
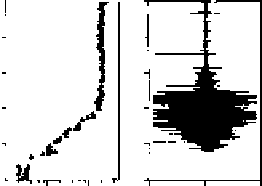
Search WWH ::

Custom Search