Geoscience Reference
In-Depth Information
the
fluids in the space, which requires interconnected
pore space.
High
formation
factor
Conductive pore
fluid in secondary
porosity
5.3.1.2
Conduction involving pore fluids
The conductivity of rocks in the uppermost few kilometres
of the crust is mainly controlled by conduction via fluids
(electrolytes) occupying pore space. It is controlled by the
type, concentration and temperature of the electrolyte;
mobility of the ions; the volume of
Conductive pore
fluid in intergranular
porosity
Increasing
complexity of
current flow
path
fluid (porosity and
degree of saturation) and its distribution (interconnectivity
and tortuosity). Note that the electrically most signi
cant
porosity in the whole-rock volume may be secondary joints
and fractures despite the fact that they may comprise only
a very small fraction of the volume. They can form excel-
lent current
flow-paths and are an important source of
electrical conductivity in fractured igneous, metamorphic
and sedimentary rocks, demonstrating that electrical con-
duction through pore
fluids is not con
ned only to porous
sedimentary rocks. The electrical properties of sedimentary
rocks, as occur in hydrocarbon basins, have been the
subject of most study, because of routine downhole logging
of electrical properties by the petroleum industry. As out-
lined below, electrical logs are a means of estimating
porosity, an important hydrocarbon reservoir property.
The resistivity of a clean porous medium (i.e. one free of
shale and clays) saturated with a saline pore fluid is empir-
ically found to be proportional to the resistivity of the
Conductive minerals
in fractures
Low
formation
factor
Figure 5.15
Schematic illustration of how pore geometry in
uences
the length and complexity of the path of electrical conduction, and
how this is related to formation factor.
3.5
5
F
=
3
f
1.44
6
8
F
=
5.9x10
-3
10
3
6
f
2.21
Planar
15
7
F
=
7
f
1.0
8.04
fluid
8
F
=
f
1.08
and so de
nes the formation resistivity factor (F), or for-
mation factor, given by Archie
10
2
4
Inter-
granular
'
s equation (Archie,
1942
)as
Vuggy
0.62
f
¼
ρ
saturated
‐
rock
a
ϕ
1
F
=
1.72
F
ρ
pore
‐
fluid
¼
ð
5
:
11
Þ
m
0.88
10
1
F
=
2
f
1.37
1
5
1.4
2
F
=
3
where
ρ
saturated-rock
is the resistivity of the fully saturated
rock and
f
1.58
0.62
ρ
ρpore-fluid
that of the saturating pore fluid (see
below for definition of
4
F
=
f
1.95
10
0
, a and m).
Formation factor is a dimensionless measure of the
passive role played by the framework of matrix material
on the overall rock conductivity. It varies between 5 and
500, with porous sandstone having a value of around 10
and poorly permeable limestone usually exhibiting a value
of several hundred. The more direct the current path, the
lower the formation factor and the closer the bulk resistiv-
ity of the rock is to the pore
fluid resistivity (F approaches
one). Formation factor is fundamentally dependent upon a
rock
ϕ
10
-3
10
-2
10
-1
10
0
Porosity (
f
)
Figure 5.16
Formation factor and porosity
fields for different types of
porosity. Also shown are a number of empirically derived
'
by Katsube and Hume (
1987
). Curves (7) and (8) are for gabbros
'
average
involving two parameters: the constant a and the cementa-
tion exponent (m). These parameters are controlled by the
nature of the pore morphology, so they are related to the
lithology and texture of the rock, and ultimately its
geological history (diagenesis etc.). Both parameters vary
s grain-scale structure (
Fig. 5.15
) and, as would be
fractional porosity (
'
). Empirical measurements show a
reciprocal relationship between F and
ϕ
ϕ
(
Eq. (5.11)
)
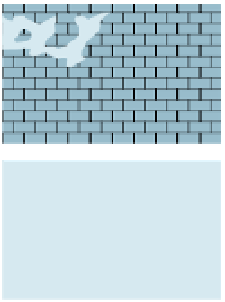

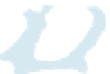



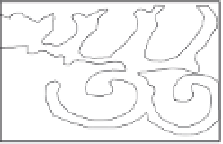
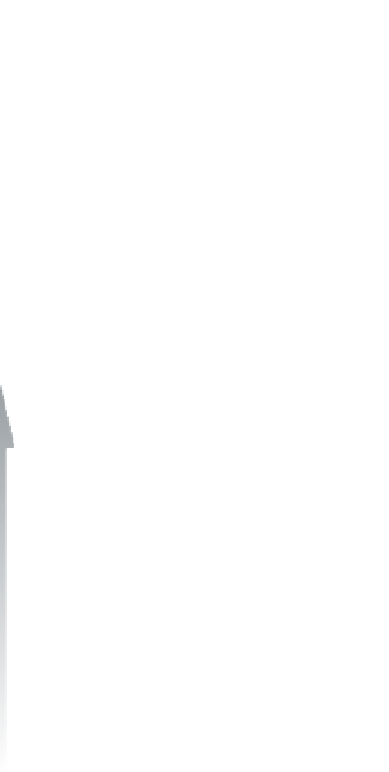
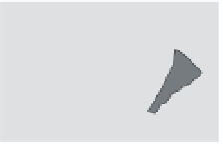






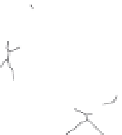









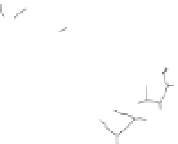











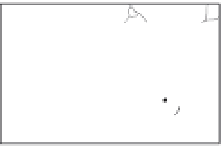







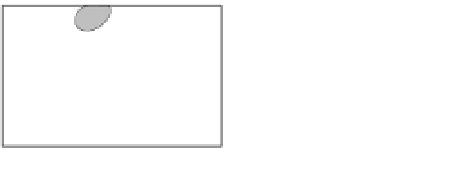


























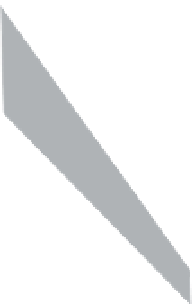
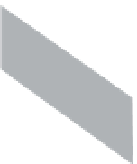

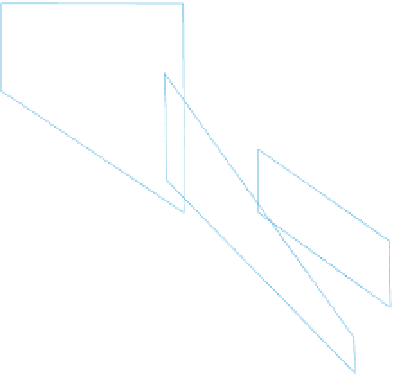
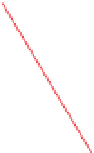
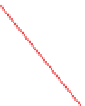
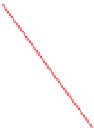
























































Search WWH ::

Custom Search