Information Technology Reference
In-Depth Information
3
Simulation Results
1.6
1x10
6
DM-DGS
DMG
SMG
DM-DGS
DMG
SMG
9x10
5
1.4
8x10
5
7x10
5
1.2
6x10
5
1.0
5x10
5
4x10
5
0.8
3x10
5
2x10
5
0.6
1x10
5
M
L1
M
L2
0
0.4
0.00
0.02
0.04
0.06
0.00
0.02
0.04
0.06
Along the lateral direction of the device (
μ
m)
Fig. 2.
Potential and Electric field of SMG, DMG, DM-DGS devices along the channel
direction near the silicon-oxide interface at V
DS
= 1 V for L =40 nm, T
si
=8 nm and T
ox
=2 nm
Fig. 2 shows the potential and electrical field distributions along the channel
direction near the silicon-oxide interface for a drain voltage (V
DS
)
= 1 V and gate
voltage (V
GS
) of 1 V. The potential distributions of DMG and DM-DGS DGJLT have
abrupt change at the workfunction transition point from W
M1
to W
M2
, whereas SMG
DGJLT follows a monotonous trend from source to drain. This enhances the electric
field of DMG and DM-DGS with two peaks, but for SMG DGJLT there is only one
peak near the drain. Out of the three devices mentioned, DM-DGS has lowest peak
near the drain, indicating that it suppresses SCE and hot carrier effect more
effectively. This is due to better gate control of DM-DGS on the channel region. The
electron velocity in the channel can be controlled by tailoring the first peak with
proper workfunction of the metal gates [11].
45
1.6
40
1.4
35
1.2
SMG
DM-DGS
DMG
30
DM-DGS
DMG
SMG
1.0
25
0.8
20
0.6
15
0.4
10
0.2
5
0.0
0
-0.2
0.0
0.2
0.4
0.6
0.8
1.0
Gate Voltage, V
GS
(V)
Fig. 3.
Transconductance (G
m
) and transconductance to drain current ratio (Gm/I
D
) with respect
to gate voltage for the devices at V
DS
= 1 V for L =40 nm, T
si
=8 nm and T
ox
=2 nm







































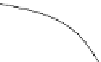














































































































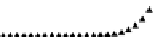

































































































































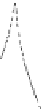













































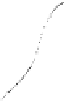







































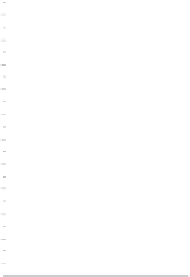















































































































































































































































