Biology Reference
In-Depth Information
In eukaryotic cells, depending on the degree
of misbalance, cells can die directly by
necrosis or, if there is damage in DNA, the
misbalance can lead to cancer. Cells have
defence mechanisms that can avoid cancer
in which cells die in a controlled way by
apoptosis (Simon
et al
., 2000). Another
mechanism of cellular defence, i.e. inflam-
mation, can worsen the scenario by increas-
ing the oxidative misbalance. Depending on
the cell type, the details of the effect caused
by the redox misbalance may change but
the general perspective of the processes are
the same.
Much work has been done in identify-
ing the main free radicals and other ROS
and NOS generated in biological environ-
ments, understanding the fine regulations of
this balance, and identifying their signalling
targets and the molecules and macromol-
ecules that could help to keep the homeosta-
sis by reacting and neutralizing ROS and
NOS (McCord and Fridovic, 1969; Halliwell
et al
., 1992). Among the ROS, singlet oxygen
(
1
O
2
) has received lots of attention recently
because its presence in several physiologi-
cal and pathological conditions was
undoubtedly demonstrated. This chapter
therefore focuses on describing some of the
properties and roles of
1
O
2
in nature and
technology, as well as describing the mol-
ecules that are known to suppress or decrease
its lifetime and consequently its effect, and
describing the mechanisms of suppression
that are involved.
Singlet
1
S
g
+
Singlet
1
D
g
Ground
3
S
g
-
Fig. 6.2.
Simplified electronic configuration of
oxygen in the triplet ground
3
S
g
+
and singlet states,
1
D
g
/
1
S
g
+
.
chain reactions (McCord and Fridovic,
1969; Halliwell, 2009).
The two electronically excited states
immediately above the ground state are both
singlet states, which are generically called
singlet oxygen (
1
O
2
). The first, designated
sigma state (
1
S
g
+
), has a very short lifetime
(less than a picosecond) and rapidly decays
to the lower singlet state (Schmidt and
Bodesheim, 1994); the other designated
delta state (
1
D
g
) has a lifetime varying from
microseconds to milliseconds in the con-
densed phase (Schmidt, 2006). Singlet oxy-
gen
1
S
g
+
lifetime in solution is so short that,
for practical purposes, it is deactivated
immediately to the
1
D
g
state.
1
O
2
(
1
S
g
+
) and
(
1
D
g
) are, respectively, 96 kJ mol
−1
(22.4 kcal
mol
−1
) and 159.6 kJ mol
−1
(38.2 kcal mol
−1
),
above the ground-state oxygen and, although
they are not directly accessible in a spin-
allowed transition from ground state, it can
be trivially formed by photosensitization by
triplet states. The triplet state of photosensi-
tizers, with energy higher than 96 kJ/mol,
can efficiently transfer energy to molecular
ground-state oxygen forming ground-state
photosensitizers and excited-state oxygen
(Abdel-Shafi and Wilkinson, 2002), as
detailed below. Living organisms use sev-
eral molecules that absorb UV-VIS photons;
therefore, photosensitization is a frequent
way in which singlet oxygen is generated in
living organisms. Because
1
O
2
has an empty
orbital, it can directly react with double
bonds, engaging in chemical reactions that
were not allowed with ground-state oxygen.
Consequently, many biological molecules
and macromolecules have evolved to pro-
tect living organisms from forming singlet
oxygen and from its effect after it has been
6.3 Singlet Oxygen: General
Properties, Detection Methods
and Biological Roles
Molecular oxygen is unusual amongst com-
mon molecules because it has an electronic
configuration that has two highest energy
electrons unpaired in the degenerated high-
est occupied molecular orbitals (HOMOs)
(Fig. 6.2). The ground-state oxygen is there-
fore a triplet state. We may expect this spe-
cies to have radical character and to react
readily with any radical species and there-
fore to facilitate the progression of radical



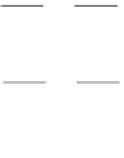









Search WWH ::

Custom Search