Biomedical Engineering Reference
In-Depth Information
The solution of the above equations are:
p
u
R
¼
J
m
ð
ur
=
c
Þ¼
J
m
ð
s
=
Tr
Þ
(7.106)
Qð
q
Þ¼
A
cos
mq
(7.107)
u
p
where
J
m
is the Bessel function of the first kind and order
m.
The
n
th zero
l
n
¼
s
=
Tr
of
u
p
J
m
ð
gives the frequency of
mn
-mode.
Figure 7.36
shows the first six vibration modes of
a circular membrane with the dimensionless frequency, which is normalized by the frequency of the
first mode (
m
s
=
Tr
Þ
2.405
f
mn
:
The number 2.405 is the first
zero of the Bessel function of zero order
J
0
. The actual vibration frequency can be determined as:
¼
0,
n
¼
1). The corresponding zeros are
l
n
¼
r
T
s
405
f
mn
2
pa
2
:
f
mn
¼
(7.108)
where
a
is the radius of the membrane. The corresponding mixing pattern with the first two modes are
shown in
Fig. 7.37
. The pattern evolves in the same time scale. Mode two has a higher frequency and
therefore induces a higher streaming velocity. The two liquids are folded and stretched leading to good
mixing in the circular chamber
[50]
.
7.7.3
Design examples
Acoustically induced flow, or acoustic streaming, is an effective transport effect for improving mixing
in microchannels. Moroney et al. reported the proof of concept of acoustic mixing in micro scale
[51]
.
The acoustic field was induced from the channel wall by flexible plate wave,
Fig. 7.38
(a). When
a flexural plate wave (FPW) propagates in a thin membrane, a high intensity acoustic field exists in the
fluid near the membrane,
Fig. 7.38
(b). This acoustic field causes net fluid flow in the direction of wave
FIGURE 7.36
The first six vibration modes of a circular membrane: (a)
f
*
¼
1.000; (b)
f
*
¼
1.594; (c)
f
*
¼
2.136;
(d) f * ¼ 2.296; (e) f * ¼ 2.653; (f) f * ¼ 2.918.
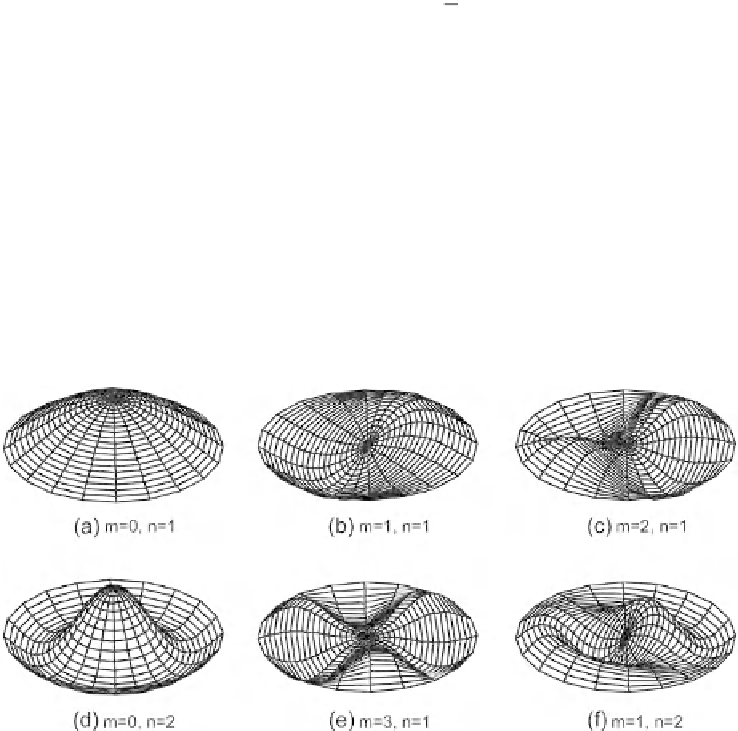




Search WWH ::

Custom Search