Biomedical Engineering Reference
In-Depth Information
The gate voltage responded with an initial spike upon illumination and then reaches a
steady-state level. Likewise, another spike of opposite polarity appeared upon cessation of
illumination. Seki et al. attributed the spike response to the intrinsic property of the ISFET
itself. However, we suspect that residual interfacial ion transfers of hR layer, even in the
absence of Cl
, may have an additional contribution. Seki et al. found a decline of the
steady-state photosignal upon the addition of Cl
. They attributed this additional change
of gate voltage to Cl
pumping of hR because it was not observed in the ISFET alone or
the PVB-coated ISFET. Nor the change could be observed by Cl
addition in the dark.
They further demonstrated that this gate voltage change is proportional to the Cl
con-
centration (Figure 15.21B).
On the basis of the foregoing discussion, the gate voltage changes were most likely
because of the interfacial Cl
transfers rather than intramolecular charge displacement of
hR. If this interpretation is correct, the performance of this hR-ISFET can be vastly improved
by using pulsed light to elicit the Cl
-dependent signal and to monitor the AC photoelectric
signal. There are several advantages for using pulsed light stimulation (cf. Figure 15.16).
First, the sensitivity of the device can be vastly enhanced. The AC photoelectric effect of
bR has a picosecond rise time, and the signal amplitude is at least two orders of magni-
tude greater than that of the DC photosignal. We suspect that the corresponding signal
from hR may have a similarly fast risetime. Second, the short duration of measurements
increases the speed of measurement. Third, the use of repeated light pulses allows for
modulation for the purpose of excluding spurious signals: the signals are measured only
when the sensor is gated by a light pulse (a technique known as gating).
Such a design can be modified to construct a light-addressable multisensor array, which
was first proposed by Haneman et al. [106]. The increased speed of measurement of AC
photoelectric signals and the use of sharply collimated or focused laser pulses make such
a design particularly attractive. Each sensor cell can then be interrogated by a scanning
pulsed laser beam in a rapid sequential fashion, thus giving an illusion of parallel
processing, much like the common technique of multiplexing in conventional microelec-
tronics. A two-sensor array based on mixed patches of hR and bR thin films illustrates
such a design (Figure 15.22). The spatially discrete patches require rapid steering of the
interrogating laser beam. If, however, the various pigments used in configuring a multi-
sensor array have nonoverlapping absorption maxima, a single sensor patch made of a
mixture of various pigments can be constructed and a rapid steering mechanism of inter-
rogating beam becomes unnecessary. Instead, a composite laser beam made of different
wavelengths must be used to interrogate the sensor patch. Even if the absorption maxima
of constituent pigments partially overlap, the composite output signal can be further ana-
lyzed and processed by means of artificial neural network technology (see Section 15.8).
In this way, investigators have a vast repertoire of known photochemistry and an equally
large variety of pigments or dyes to exploit for design purposes.
15.7
Bioelectronic Interfacing
In the examples presented in Section 15.6, the photoelectric signal is capacitance-coupled
(AC-coupled) to the metal electrode. The devices pose no interfacing problem in hooking
up with conventional microelectronic circuitry for further signal processing. However, a
number of investigators have found that no DC photoelectric current can be observed in
bR-coated metal-electrode sensor systems. This was most likely due to the absence of
appropriate electrodic reactions in the system to convert a current carried by protons in

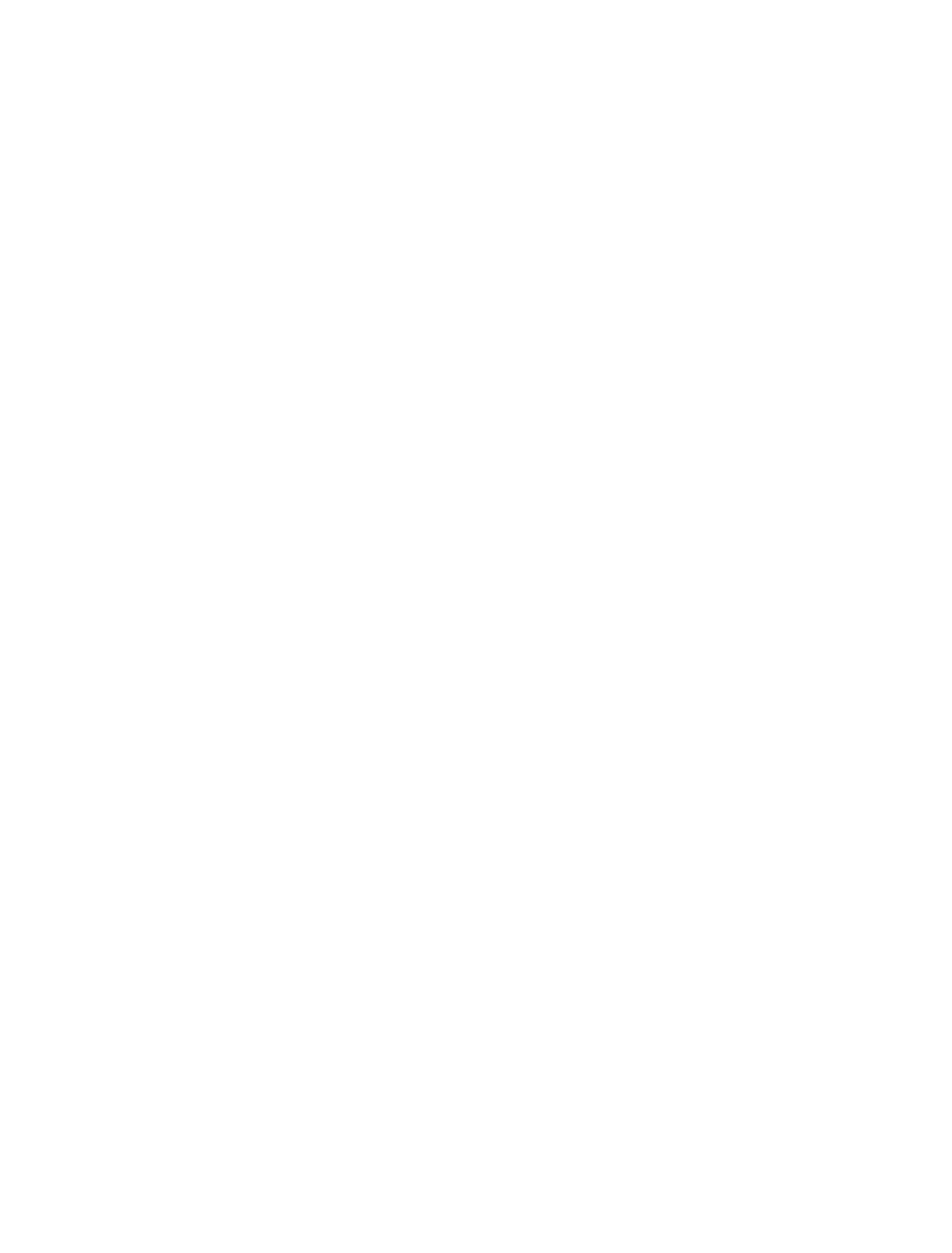