Biomedical Engineering Reference
In-Depth Information
2.4.3 R
OBOT
-A
SSISTED
C
ONSTRUCT
F
ABRICATION
A small number of groups work on this type of technology. This work was reviewed by Zhang
et al.
42
The principle of microassembling a scaffold/cell construct is based on the same concept of
assembling a structure using small building block units like Lego (Figure 2.11). Building blocks of
different designs are fi rst fabricated via lithography or microfabrication technologies and are then
assembled by a dedicated precision-robot with four degrees of freedom microgripping capabili-
ties, accomplishing a functional-sized scaffold with the required material, chemical, and physical
properties. A monolithic shape memory alloy microgripper was used to manipulate and assemble
the unit microparts into a scaffold structure. Even though preliminary data are promising, future
studies need to show if this technique can be translated into clinically applicable concepts.
2.5 CONCLUSIONS
Scaffolds are of great importance for tissue engineering because they enable the fabrication of
functional living implants utilizing cells directly obtained from cell culture. As the scaffolds for
tissue engineering will be implanted in the human body, the scaffold materials should be nonanti-
genic, noncarcinogenic, nontoxic, nonteratogenic and possess high cell/tissue biocompatibility so
that they will not trigger pathological reactions after implantation. Along with stringent material
considerations, the macro and microstructural properties of the scaffold are also very important. In
general, the scaffolds require specifi c individual external shapes and well-defi ned internal structure
with interconnected porosity to host most cell types. From a biological point of view, the designed
matrix should serve several functions, including (1) the ability to act as an immobilization site for
transplanted cells, (2) the formation of a protective space to prevent unwanted tissue growth into
the wound bed and allow healing with differentiated tissue, (3) directing the migration or growth
of cells via surface properties of the scaffold, and (4) directing the migration or growth of cells via
release of soluble molecules such as growth factors, hormones, and cytokines. Future work has to
provide further compelling evidence that SFF offers the right balance of capability and practicality
to be suitable for fabrication of materials in suffi cient quantity and quality to move holistic tissue
engineering technology platforms into a clinical application.
In addition to considerations of scaffold performance based on tissue engineering strategies,
practical considerations of manufacture also arise. From a clinical point of view, it must be possible
to manufacture scaffolds under good manufacturing practice (GMP) conditions in a reproducible and
quality-controlled fashion at an economic cost and speed. To move the current tissue engineering
practices to the next frontier, some manufacturing processes will be required to accommodate the
incorporation of cells and growth factors during the scaffold fabrication process. To address this issue,
novel manufacturing processes such as robotic assembly and machine- and computer-controlled
FIGURE 2.11
(A) Simple building blocks (
left
) were manually fabricated to illustrate the original concept
for microassembly concept. The feasibility of designed parts was studied by using parts made 10
×
larger
than the original parts (
right
). (B) A group at the National University of Singapore started developing a novel
advanced manufacturing technique to fabricate scaffold/cell constructs for various tissue engineering applica-
tions in 2001. The idea was to assemble microscopic Lego-like building blocks into a scaffold. Based on this
CAD-based and automated concept, the distribution of growth factors and living cells within the scaffold can
be truly controlled in three dimensions so that scaffold/cell constructs with customized biological and physi-
cal properties can be realized. (C) The fabrication of these microparts is challenging due to their small size
(0.5 µm
×
0.5 µm
×
0.2 µm overall, 60 µm thickness,
±
5 µm tolerance) and complex 3-D shape. To facilitate
the microassembly process, another challenge is the requirement that the parts need to be stably fi xed on the
wafer but at the same time are able to easily be removed by the microgripper. Currently, SU-8, a biocompatible
material, is used to test the feasibility of the microassembly. (From Zhang, H., Hutmacher, D.W., Chollet, F.,
Poo, A.N., Burdet, E. Review Macromol. Biosci. 5, 477-489, 2005. With permission from Wiley.)

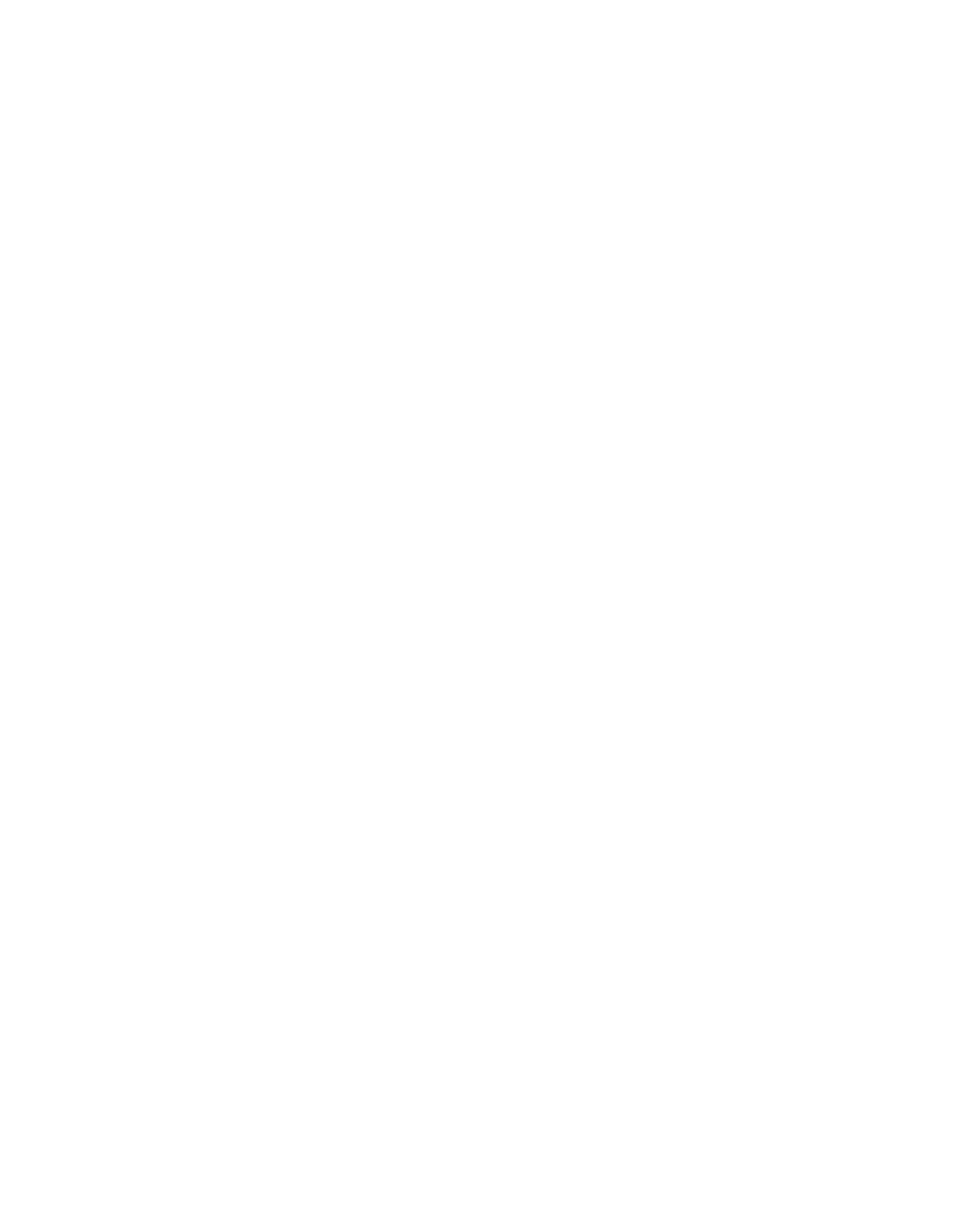