Biomedical Engineering Reference
In-Depth Information
Cells
Solvent casting and particulate leaching
Sub-pores
Sub pores
Pores
Pores
Gas foaming
Emulsification and phase separation
Fiber diameter
Electrospinning
Casting
Tube diameter
Nanopattern
Lithography
Solid free-form fabrication
Pores
Pores
FIGURE 2.6
SFF fabrication techniques compared to other scaffold fabrication technologies and the dimen-
sions of the generated scaffolds.
where the laser beam strikes the surface of the bath, resulting in the creation of a fi rst solid plastic
layer at and just below the surface. The solid layer is then lowered into the bath, and the laser-
generated polymerization process is repeated for the generation of the next layer, and so on, until
a plurality of superimposed layers forming the desired scaffold architecture is obtained. The most
recently created layer in each case is always lowered to a position for the creation of the next layer
slightly below the surface of the liquid bath. Once the scaffold is complete, the platform rises out of
the vat and the excess resin is drained. The scaffold is then removed from the platform, washed of
excess resin, and then placed in an UV oven for a fi nal curing.
For industrial applications, the photopolymer resins are mixtures of simple low-molecular-weight
monomers capable of forming solid long-chain polymers by chain reactions when activated by radi-
ant energy within specifi c wavelength range. The commercial materials used by SL equipment are
epoxy- or acrylate-based resins that offer strong, durable, and accurate parts or models. However,
these materials cannot be used as scaffold materials due to the lack of biocompatibility and biodegrad-
ability. Hence, the limited availability of photopolymerizable biomaterials is a major constraint for the
use of the SL technique in the design and fabrication of scaffolds for tissue engineering applications.
However, biocompatible acrylic, anhydride, and polyethylene oxide (PEO)-based polymers may be
explored in future research, as they are already typically at the clinical research stage as curable bio-
adhesives or injectable materials. A variation in laser intensity or traversal speed may be employed
to alter the cross-link or polymer density within a layer so that the properties of the material can be
varied from position to position within the scaffold. This variation enables the fabrication of so-called
biphasic or triphasic matrix systems. Microstereolithography (MSL) in particular is thought to offer a
great potential for the production of 3-D polymeric structures with micrometer resolution.
2.3.1.2
Selective Laser Sintering
Selective laser sintering (SLS) also uses a focused laser beam, but with the intention of sintering
areas of a loosely compacted powder. In this method, a thin layer of powder is spread evenly onto
a fl at surface with a roller mechanism. The powder is then raster-scanned with a high-power laser
beam. The powder material that is struck by the laser beam is fused, while the other areas of powder
remain dissociated. Successive layers of powder are deposited and raster-scanned, one on top of one
another, until an entire part is complete. Each layer is sintered deeply enough to bond it to the pre-
ceding layer. Wiria et al. have used SLS to produce biocompatible PCL/TCP scaffolds from starting
powders; the scaffold demonstrated good osteoblast-like cell responses such as attachment levels
and proliferation.
23
However, SLS has inherent material constraints for scaffold fabrication and is



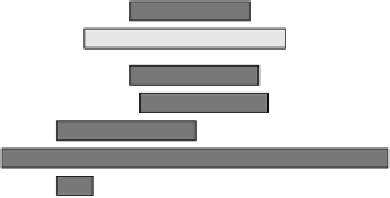







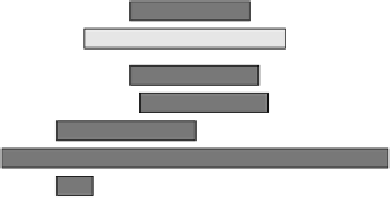




