Geography Reference
In-Depth Information
Figure 4.3. Travel time distributions
at the catchment outlet when
considering only short flow paths
on the hillslope and in the stream
network (a, c) and longer flow paths
through deeper groundwater (b, d).
From Frisbee et al.(
2011
).
While soils and soil structure (connected vertical and
lateral preferential pathways) strongly control the dynam-
ics of relatively fast responding local flow paths with time
scales ranging between days and years, geology controls
deeper flow paths that are commonly active on the regional
scale and at time scales from years to decades (Gleeson and
Manning,
2008
; Möller et al.,
2007
). The active flow paths
and residence times depend strongly on the aquifer struc-
ture (Kupfersberger and Blöschl,
1995
). For example,
Schaller and Fan (
2009
) and Fan et al.(
2007
) showed that
geological discontinuities such as fault lines are a first-
order control of the direction of flow in deep flow paths.
Flow paths are highly sensitive to the strata dip angle, and
travel times can thus differ by a factor of up to two at
opposite stream banks due to strata dip angles. Karst flow
systems tend to be highly dynamic and the connection with
surface water may be a function of the groundwater levels
(e.g., Bonacci et al.,
2008
; Filipponi et al.,
2009
). The
response characteristics then depend on the relative contri-
butions from the matrix, fracture and conduit system
significantly, which will be the more complete representa-
tion of the subsurface flow system. Flow paths can be
grouped into
where geology, climate,
vegetation and water table conditions are distinct (Duffy,
2004
): shallow water tables in steep upland hillslopes over
bedrock, deep water tables and shallow water tables in the
alluvium. Even shallower bedrock can convey substantial
water fluxes, as illustrated by Anderson et al.(
1997
) for a
steep catchment in the Oregon Coast Range.
'
similarity zones
'
4.3 Inference of flow paths and storage from
response characteristics
4.3.1 Inference from runoff
Learning from temporal patterns of runoff in one
catchment
Analysis of the observed runoff dynamics can be used to
infer catchment storage and subsurface characteristics by a
top-down approach (Tallaksen,
1995
). The classic method
is runoff recession analysis. The main advantages of reces-
sion analyses are that rainfall can be assumed to be zero, or
at least small (so difficulties with any errors in catchment
rainfall estimation are avoided), and that the hydrograph
represents an aggregate measure of catchment behaviour
(Sivapalan et al.,
2003b
). There are two classes of methods
for interpreting the runoff recession. The first class is based
on empirical storage
-
the
preferential flow network in the Karst
leading to vastly
contrasting time scales of catchment response (Florea and
Vacher,
2007
). Karst aquifers can also lead to significant
subsurface water transfers between catchments (e.g.,
Quinn et al.,
2006
). The importance of considering both
shallow and deep flow paths has been illustrated by Frisbee
et al.(
2011
). In a simulation study they analysed the
contribution of different types of flow paths to the travel
time distribution at the catchment outlet (
Figure 4.3
).
Clearly, including longer flow paths through the deeper
groundwater system increases the estimated travel times
-
runoff relationships and/or on con-
ceptualising the catchment as a set of linear or non-linear
reservoirs. In the most basic method, the integral under an
exponential curve fitted to the runoff recession curve
-
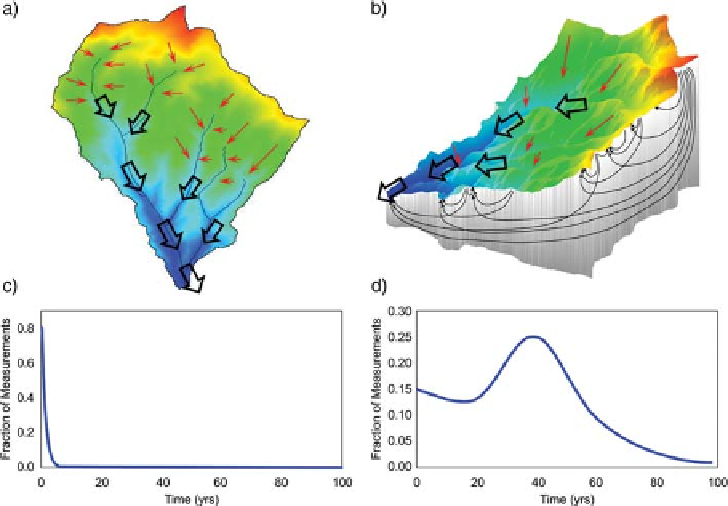
Search WWH ::

Custom Search