Environmental Engineering Reference
In-Depth Information
12.8.2 Frequency and Sensitivity Analysis
The assessment of weather-related geohazards is undertaken
in terms of the frequency distribution as a measure of
stability (i.e., the factor of safety). The choice of variables
that must be modeled as uncertainties (i.e., probabilistically)
is determined by performing a sensitivity analysis. The
decision analysis framework adopted in the weather hazard
model provides a systematic environment for frequency
and sensitivity analyses.
The frequency analysis corresponds to one step in the
decision analysis cycle. In order to perform frequency
analyses, the relationships, dependences (or correlations),
and frequency distributions of the involved parameters
must be established. These relationships can be illustrated
using an influence diagram for the weather hazard model
(Fig. 12.118). The variables presented inside round boxes
are the uncertain variables. The variables presented inside
square boxes are certain (or fixed) values. These values
are part of the deterministic equations and do not need to
be shown on the influence diagram. The arrows indicate
the relationships between variables. The factor-of-safety
box is the final outcome receiving arrows from outside.
Only some of the incoming variables need to be modeled
as uncertainties. These variables are determined from
sensitivity analyses performed on all the variables involved
with assessing stability. A discrete stochastic analysis can be
used to perform a frequency analysis.
The presence of several uncertain variables produces a com-
bination of different scenarios. The presentation of several
scenarios is called the decision tree. Figure 12.119 presents
the decision tree corresponding to the influence diagram of
Fig. 12.118. Three branches are used for each variable. The
notation used for the decision tree is the same as that used
1.2
90
High
hazard level
80
1.15
70
Factor of safety
Intermediate
hazard level
60
50
1.1
40
30
Low
hazard level
1.05
20
SWCC
10
0
1
0.1
1
10
100 1,000 10,000 100,000
10
6
Soil suction, kPa
Figure 12.117
SWCC as water level gauge and embankment haz-
ard gauge.
the soil suction is given by the SWCC (Fig. 12.116). Typical
SWCCs can be defined by three primary soil parameters: air-
entry value
ψ
aev
, residual suction
ψ
r
, and residual degree of
saturation
S
r
(Gitirana and Fredlund, 2003b).
Figure 12.117 presents an analogue describing the funda-
mental concept behind the weather hazard model. According
to this analogue, the soil comprising an embankment can
be viewed as a “water tank.” The SWCC acts as a gauge
that indicates the water level within the water tank. The
embankment factor of safety
F
s
and the embankment haz-
ard level vary according to the water level. A low water
level corresponds to a lower level of hazard (i.e., higher
F
s
),
while higher water levels produce greater hazards (lower
F
s
). The model for assessment of embankment hazards is
based indirectly on the water tank concept and the factor
of safety. However, a more approximate approach could be
used to indicate the water level within the water tank. Such
an approach would still capture the main factors controlling
the stability of an embankment or slope.
Saturated
hydraulic
conductivity
Potential
evaporation
Relative
hydraulic
conductivity
Initial
pore-water
pressure
distribution
Precipitation
Vapor
diffusion
D
vap
SWCC:
Residual
saturation
Geometry
Pore-water
pressure and
temperature
distributions
Water
strage:
m
2
w
Shear
strength
parameter
SWCC:
Residual
suction
Thermal
properties
SWCC:
Air-entry value
External
load
Stress
distribution
Factor of
safety, F
s
Figure 12.118
Influence diagram for weather hazard model.













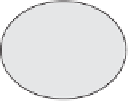







Search WWH ::

Custom Search