Biomedical Engineering Reference
In-Depth Information
Fig. 13.4
Coupled excitation-induced contraction of generic heart model. Snapshots of the de-
formed model depict the action potential contours at different stages of depolarization. The
yellow
lines
denote the spatial orientation
f
of contractile myofibers. The two slices in the translucent
images in the
lower row
favorably depict the wall thickening and the twisting motion of the heart
13.5 Conclusion
In this contribution, we have proposed a new kinematic approach to the compu-
tational cardiac electromechanics. For this purpose, the deformation gradient has
been multiplicatively decomposed into the active and passive parts. The evolution
of the former has been considered to be function of the transmembrane potential.
In addition, the inherently anisotropic, active architecture of cardiac tissue has been
accounted for within the proposed kinematic framework. As opposed to the active-
strain formulations proposed in the literature, the proposed formulation results in
the additively decomposed stress response, which has been attributed to the active-
stress formulations only. The proposed micro-structurally based kinematic approach
to electro-active materials is believed to be advantageous over the entirely stress-
based formulations, since the active deformation is more readily accessible through
most experimental techniques at different scales. Therefore, the material parameters
governing the generation of the active straining upon electrical stimulation can be
favorably tuned to yield reliable, predictive simulations. The proposed kinematic
setting has then been embedded in the fully implicit, entirely finite-element-based
coupled framework. The implicit numerical integration of the transient terms and the
monolithic solution of the resultant coupled algebraic equations has led us to an un-
conditionally stable and modular structure. The performance of the proposed model
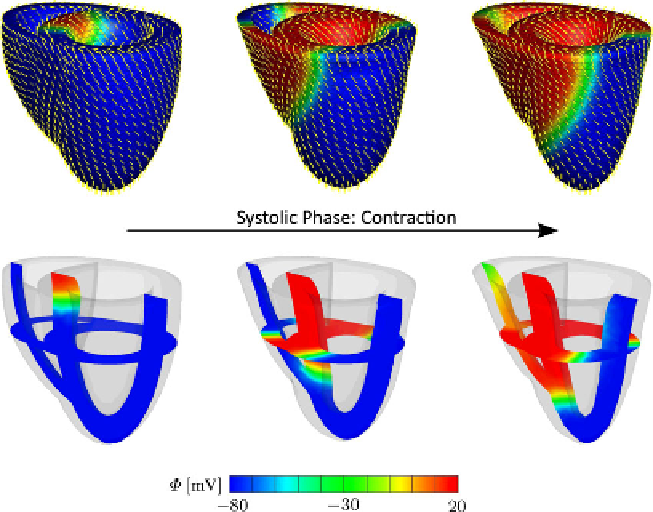