Biomedical Engineering Reference
In-Depth Information
microscopic level, cell growth and differentiation and
ultimate tissue formation are dependent on mechanical
input to the cells. As a consequence, the scaffold must
be able to both withstand specific loads and transmit
them in an appropriate manner to the surrounding
cells and tissues. Specific mechanical properties of
scaffolds include elasticity, compressibility,viscoelastic
behavior, tensile strength, failure strain, and their time-
dependent fatigue.
because the degradation of material is a result of enzy-
matic, biological action. When a material disappears by
being taken into another body, we call the phenomenon
''absorption''. In this case a decrease in MW is not a nec-
essary condition. A good example for this is alginate
which is water soluble but becomes water insoluble upon
addition of divalent cations such as Ca
2
þ
. This water-
insoluble gel recovers to a water-soluble sol when a high
concentration of monovalent, sodium ion is present. If
this sol-gel transition takes place through ion exchange in
the living body, one can say that the alginate-Ca
2
þ
complex has been ''absorbed'' into the body without a
decrease in MWof the starting alginate. As this example
suggests, the term ''absorbable'' (or resorbable) seems to
be more suitable than ''degradable'' so far as their medical
use is concerned, because absorption includes both
chemical degradation (either by passive hydrolytic or by
enzymatic cleavage) and physicochemical absorption
(through simple physical dissolution into aqueous
media). Here the term ''absorbable'' (or ''bioabsorbable'')
will be mostly used. The term ''bioabsorbable'' is used
simply because the absorption proceeds in the biological
environment.
Specific bulk and surface properties
7.2.2 Absorbable biomaterials
Biomaterials are a critical enabling technology in tissue
engineering, because they serve in various ways as a sub-
strate on which cell populations can attach and migrate,
as a 3-D implant with a combination of specific cell types,
as a cell delivery vehicle, as a drug carrier to activate
specific cellular function in the localized region, as a me-
chanical structure to define the shape of regenerating
tissue, and as a barrier membrane to provide space for
tissue regeneration along with prevention of fibroblast
ingrowth into the space. In many cases a biomaterial
serves a dual role as scaffold and as delivery device.
Degradation and absorption of biomaterials are essential
in functional tissue regeneration, unless the application is
aimed at long-term encapsulation of cells to be immu-
nologically isolated. Materials that disappear from the
body after they have fulfilled their function obviate
concerns about long-term biocompatibility. The by-
products of degradation must be non-toxic, similar to the
starting material. For a biomaterial to be accepted in the
medical system, its safety and efficacy must be proven
with any therapy. Ideally, the rate of scaffold degradation
should mirror the rate of new tissue formation or be
adequate for the controlled release of bioactive mole-
cules.
Table 7.2-1
represents naturally occurring and
synthetic biomaterials that possess hydrolysable bonds in
the main chain. Their current medical applications are
summarized in
Table 7.2-2
. Clinical applications of ab-
sorbable biomaterials have a long history similar to those
of non-absorbable biomaterials. Absorbable sutures like
catgut and hemostatic or sealing agents like collagen,
oxidized cellulose, and a-cyanoacrylate polymers have
been the front runners in the medical use of absorbable
biomaterials. The largest clinical application of absorb-
able biomaterials at present is for suturing and ligature.
The technical term ''degradable'' that has been used
for biomaterials has several synonyms including bio-
degradable, absorbable, bioabsorbable, and resorbable. In
general, the term ''degradable'' is used when the molec-
ular weight (MW) of the polymer constructing the ma-
terial does decrease over time. When such degradation
takes place only in a biological environment where en-
zymes exist, the term ''biodegradable'' is preferably used
including me-
chanical strength, absorption kinetics, wettability, and
cell adhesion
d
are required for the biomaterials that are
used for tissue engineering. Large numbers of both bi-
ologically derived and synthetic materials that meet these
requirements have been extensively explored in tissue
engineering. These biomaterials can be categorized ac-
cording to several schemes. Here their categorization is
based on their source.
d
7.2.2.1 Natural polymers
The origin of naturally occurring polymers is human,
animals, or plants. Materials from natural sources such as
collagen derived from animal tissues have been consid-
ered to be advantageous because of their inherent prop-
erties of biological recognition, including presentation
of receptor-binding ligands and susceptibility to cell-
triggered proteolytic degradation and remodeling. How-
ever, the biologically derived materials have concerns,
especially complexities associated with purification, sus-
tainable production, immunogenicity, and pathogen
transmission. Apart from this fact, medical applications
of absorbable natural polymers are limited, because their
mechanical strength is not strong enough when hydrated.
One exception is chitin (and chitosan) that is a crystalline
polymer. Most of natural polymers are soluble in aqueous
media or hydrophilic. Because water-soluble polymers
are not appropriate as a 3-D scaffold, they should be
converted into water-insoluble materials by physical or
chemical reactions.

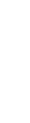




