Hardware Reference
In-Depth Information
I
B2
−L
I
B1
X Z−
DO-CCCII
I
1
I
2
I
1
V
1
Z−
DO-CCCII
X
I
2
V
2
Z+
V
1
V
2
Y
Z+
Y
C
Fig. 9.22 Current-controlled floating negative inductance
capacitance. By a straight forward analysis, the circuit of Fig.
9.23a
has the input
impedance given by:
Z
1
Z
2
R
x
3
Z
in
¼
R
x1
þ
R
x2
þ
ð
9
:
23
Þ
From this expression, it is seen that with Z
1
¼
R
2
, the circuit can
realize a resistance in series with a grounded capacitor whose value is
electronically-controllable by the external current I
B3
. On the other hand, with Z
1
and Z
2
both taken as capacitors, the circuit would realize a resistance in series with
a grounded FDNR whose value would be controllable by I
B3
.
A floating capacitance multiplier employing grounded capacitor is shown in
Fig.
9.23b
. This circuit simulates a floating capacitance in series with a resistance
with parameter values as
C
eq
¼
1/sC
1
and Z
2
¼
C
1
R
x
4
(
R
x
1
+
R
x
2
).
Synthetic transformer may be useful element for constructing stagger-tuned
filters. Compared to passive transformers, an active transformer provides the
advantages of integratability, easy adjustment of the coupling co-efficient and
elimination of any magnetic interference (because they do not employ any induc-
tive component). A circuit using CCCIIs, to simulate a synthetic transformer,
derived from a circuit proposed by Yuce and Minaei [
21
], is shown in Figs.
9.24
and
9.27
This circuit is equivalent to an inductive-T network with two self-inductances L
1
and L
2
and mutual inductance M such that the two ports can be modeled by the
following matrix equation:
R
x
3
and
R
eq
¼
¼
I
1
I
2
V
1
V
2
sL
p
sM
12
ð
9
:
24
Þ
sM
21
sL
s















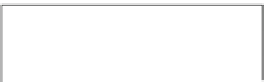




















































Search WWH ::

Custom Search