Environmental Engineering Reference
In-Depth Information
When
Q
i
equals the required demand,
Q
req
, the value for
H
i
should equal the desired head,
H
des
, in the node. It is the head that should be available if the demand at that node is to be
satisfied in full. Hence:
1
/
n
des
i
min
req
i
H
−
H
1
Q
req
i
3.4
Q
=
i
⇒
=
(
)
n
1
/
n
1
/
K
K
des
i
min
H
−
H
i
i
i
Finally, substituting
K
i
in Equation 3.3 yields:
1
/
n
H
avl
i
−
H
min
avl
i
req
i
3.5
Q
=
Q
i
des
i
min
H
−
H
i
where
Q
i
avl
is the discharge available for the head available at the node (
H
i
avl
). Equation 3.5
considers three possible situations:
1.
avl
i
min
avl
i
H
≤
H
⇒
Q
=
0
i
2.
min
avl
des
i
avl
i
req
i
H
<
H
<
H
⇒
0
<
Q
<
Q
i
i
3.
avl
i
des
avl
req
i
H
≥
H
⇒
Q
=
Q
i
i
and as such it is used in balancing of the flows in the pipes connected to node
i
. The solution
algorithms for solving the system of head equations have been described by Gupta and Bhave
(1996). Eventually, the head-driven simulation is able to determine the nodes with
insufficient supply. Apart from more complex and longer simulation, the key problem here is
the correct definition of the values for
H
i
min
and
H
i
des
head, i.e. their correlation to the nodal
resistance
K
i
, which describes the nature of the PDD relationship that is essentially empirical.
Figure 3.4 shows the approximate PDD relation from the Dutch experience (KIWA, 1993). A
linear relation is suggested until a certain threshold, which is typically a pressure around 20
mwc. In this way of presentation, the critical pressure
p
i
crit
/ρg
=
H
i
des
- H
i
min
.
Figure 3.4
Pressure-related demand relation (KIWA, 1993)
The concept of EPANET emitter coefficients uses similar relationship as in Equation 3.2. An
emitter is modeled as a setup of a dummy pipe connecting the actual node with a dummy
reservoir whose initial head equals the nodal elevation,
z
. Hence,
H
i
min
= z
i
and:


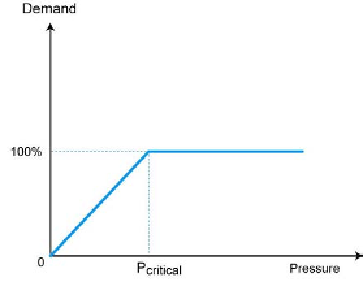

Search WWH ::

Custom Search