Geoscience Reference
In-Depth Information
algae, bacteria or the atmosphere. It consists
mainly of goethite or limonite (HFeO
2
) in spongy,
porous, impure deposits often mixed with plant
debris and other sediments.
4.6 Summary
Water quantity and quality are essential ingredi-
ents for all environments and life on Earth.
Hydrology is the science of water, and wetlands
represent a storage point within the hydrologic
cycle. Wetlands may gain water from direct pre-
cipitation, surface inl ow, and ground-water
discharge; water is lost by evapotranspiration,
ground-water recharge, and surface outl ow.
Water storage capacity, hydroperiod, and resi-
dence time vary greatly for different kinds of
wetlands; some are quite stable through time,
others change regularly or erratically.
Many wetlands are hydrologically connected
with underlying ground water, which moves in
response to the hydraulic gradient at rates deter-
mined by hydraulic conductivity and hydrostatic
pressure. Some wetlands are sustained by ground-
water discharge; in other cases, wetlands may
recharge aquifers. Human diversions or extrac-
tions of either surface l ow or ground water
have the potential to impact integrated surface-
ground-water systems. Overpumping ground
water and diverting l ow from surface streams
are among the common means by which people
adversely affect wetlands both locally and
downstream.
Flooding is a universal phenomenon that
affects many wetlands in all climatic regimes
and any size or type of drainage basin. Flooding
is an entirely natural event that takes place on
most streams every few years. However, l ood-
ing becomes a hazard when high water leads
to human casualties, damage to structures, and
impairment of human land use. Most types of
human land use and development lead to
increased frequency and magnitude of l ooding,
because the l ood-moderating inl uence of
natural wetlands is reduced.
Water chemistry involves both organic and
inorganic aspects that are affected by diverse
natural processes and human activities. Natural
Figure 4-21.
Turner Falls, south-central Oklahoma,
United States. The falls are developed on massive tufa,
which forms the cliffs on either side. The tufa
precipitated from hard spring water derived from
ground water in thick limestone and dolostone bedrock
of the Arbuckle Mountains. Photo by J.S. Aber.
Fe
2
+
(
ferrous
)
=
Fe
3
+
(
ferric
)
+
e
−
=
(
Eh
077
.
volts
)
Furthermore, iron is usually present along with
other dissolved solids and gases in natural waters,
which gives rise to more complex reactions. Con-
sider, once again, the case of dissolved carbon
dioxide:
2
Fe
2
+
+
−
+
+
=
+
4
HCO
H O
O
2
Fe OH
(
)
4
CO
1
2
3
2
2
3
2
It is clear that both oxygen (Eh) and carbon
dioxide (pH) control this reaction toward ferrous
(left) or ferric (right) states. This situation is
illustrated with an Eh-pH diagram, also known
as Pourbaix diagram, which plots Eh on the
vertical axis and pH on the horizontal axis
(Fig. 4-22). Wetlands are places where reducing
conditions often develop, particularly within
saturated soils, so the status of iron is a critical
indicator (see chapter 5). Eh and pH may be
measured quickly in the i eld using portable
instruments in order to establish the chemical
environment.
Ground water often contains considerable
dissolved iron in the ferrous state. When such
ground water emerges in springs, it comes into
contact with air, is rapidly oxidized, and iron
precipitates as insoluble ferric deposits, namely
rust (Fig. 4-23). Bog iron ore forms in a similar
way, when iron-bearing water is oxidized by


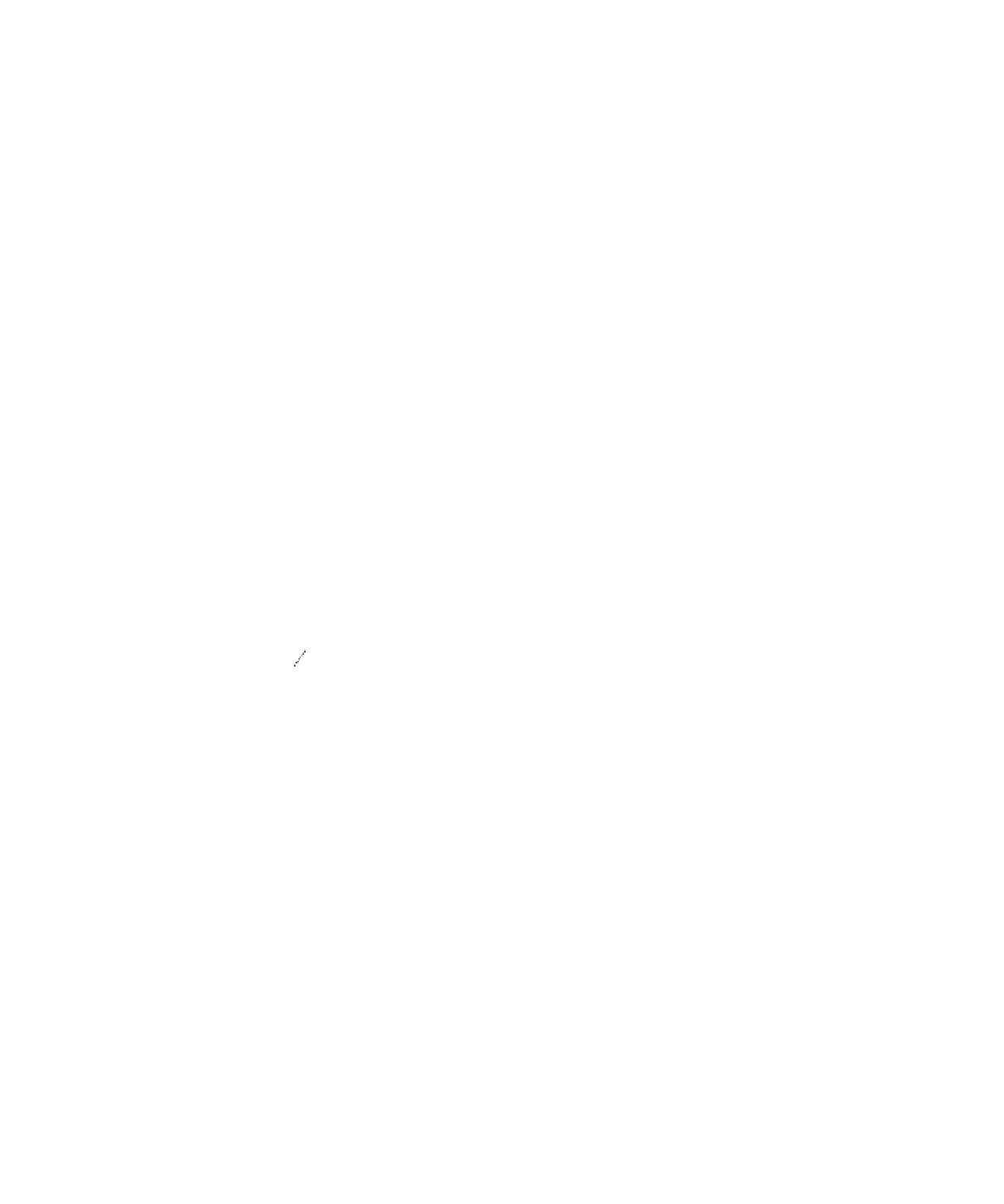
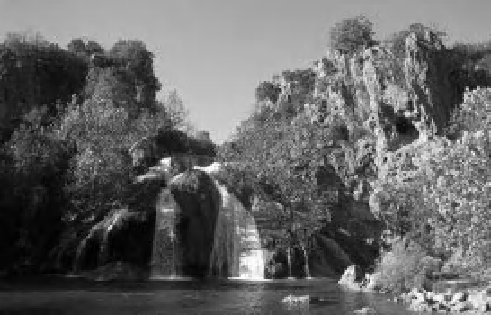


