Chemistry Reference
In-Depth Information
O
OH
Ph
OMe
Ph
O
O
MeO
O
O
[G4]
UV, DMPA
+
[G4]
OH
HS
R
O
R=
O
S
R
48
Solvent free or THF/DMF
HN
48
Fmoc
O
O
scheme 2.13
Modification of dendrimer g4-ene
48
using thiol-ene radical reaction.
MesN
NMes
Cl
Ru
Cl
i
PrO
+
R
R
S
S
Water-
t
BuOH, pH 8.0
2-5 h, rt to 37°C
Target protein
Target protein
scheme 2.14
Protein modification using olefin cross metathesis.
Recently, thiol-ene chemistry has been used on the cell surface of living cells, which metabolically present methacryloyl
groups [199]. After uV exposure for 10 minutes in the presence of a photoinitiator (Irgacure 2959 or biMA), thiol-Peg
could be introduced to the cell surface. Although no cytotoxicity was reported in the paper, thiol-ene chemistry, at its current
stage, does not seem to be an optimal chemistry for use in cells or living systems.
Interestingly, electron-deficient alkynes, such as alkynoic amides, esters, and alkynones, could react with cysteine-containing
peptides or proteins in aqueous media to form reversible modifications [200]. Modification of unprotected peptides and proteins
occurred via the formation of a vinyl sulphide linkage, which could be cleaved off by addition of thiols under mild conditions.
2.2.3.3 Cross Metathesis
olefin metathesis is a great tool for building molecular architectures in organic synthesis
[201-203]. Among various types of olefin metathesis, cross-metathesis is not as straightforward or efficient as ring-closing
metathesis and ring-opening metathesis polymerisation due to the lack of the entropic driving force existing in RCM and
side reactions arising from self-metathesis. development of metathesis catalysts that can be used in aqueous media greatly
facilitated theirs application to modify biomolecules in water or water-organic solvent mixtures [204-206]. Protein modifi-
cation using cross metathesis was first realised by the davis group, after chemical introduction of alkene groups to cysteine
thiols on proteins (Scheme 2.14) [207]. Addition of a magnesium salt in the aqueous-alcohol media in the presence of a
ruthenium catalyst (Hoveyda-grubbs second generation) allowed the reactions to be completed at pH 8 at 37°C with high
efficiency, and various groups, such as alcohols, carbohydrates, and oligoethyleneglycol, could be introduced to a protein
surface. It was also noticed that allyl chalcogenides generally enhance the rate of alkene metathesis reactions, and allyl sel-
enides were found to be exceptionally reactive olefin metathesis substrates, enabling a broad range of protein modifications
[208]. Although not a direct conjugation strategy, ring-opening metathesis polymerisation could generate hydroxypyrido-
nate-type gadolinium chelators, built in polymer backbones [209]. These chelates exhibited extremely large molecular relax-
ivities (r
1
> 100 mM
-1
s
-1
), presumably due to the longer rotational correlation times estimated from the molecular weights of
linear polymers.
2.2.4
cross-coupling reactions
Cross-coupling reactions, such as those in synthesis of natural products and construction of polymers [210, 211], have been
applied extensively in organic synthesis. In molecular imaging, cross-coupling reactions are also used in the preparation of
radiotracers [212]. However, their use in conjugation or labelling of biomolecules is limited by low conversion rates and
harsh conditions with the use of transition metal catalysts, which may denature the biomolecules. nonetheless, by carefully
modifying reaction conditions, a few groups have extended the cross-coupling reactions in protein labelling or modification.
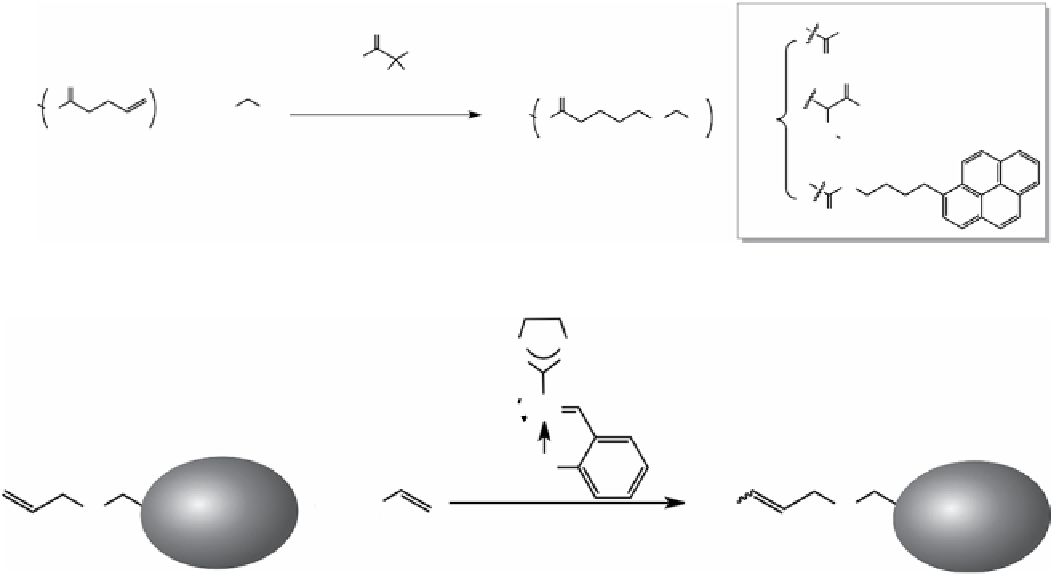