Chemistry Reference
In-Depth Information
1.6
WhAt Is oPtIcAl IMAgIng?
optical imaging techniques exploit different properties of light, such as absorption, emission, reflectance, scattering, polari-
sation, coherence, and fluorescence, as a source of contrast. Such contrast is created by the interaction of photons with differ-
ent tissue or cellular components. Fluorescent and luminescent properties of light are generally used in
in vivo
studies.
Therefore, this section will focus on fluorescence imaging. Although other optical imaging systems such as bioluminescence
imaging, optical coherence tomography, photoacoustic microscopy, and tissue spectroscopy are not mentioned here (but are
briefly discussed in chapter 11), this does not mean that they play a less significant role in the development of optical imaging.
Fluorescence-based techniques are extremely valuable for studying cellular structures, functions, and pathways as well
as molecular interactions in biological systems. These techniques utilise microscopy systems to observe and image at the
microscopic and macroscopic levels, as well as molecular labels/dyes for contrast enhancement [30]. There are numerous
types of labels and dyes for optical imaging; one example of a classic dye is shown in Figure 1.18.
1.6.1
Basic Principles
Fluorescence results from a process that occurs when certain molecules absorb light. These molecules are generally polyaro-
matic hydrocarbons or heterocycles known as fluorophores. They absorb energy at a particular wavelength and emit energy
at a different but specific wavelength. This can be explained by a simple electronic state Jablonski diagram (Figure 1.19).
The electronic states of most organic molecules are either singlet states (S
1
), when all electrons in the molecule are spin-
paired, or triplet states (T
1
), when one set of electron spins is unpaired. Upon excitation, the molecules move to a temporary
excited state S
1
, which can relax by numerous decay mechanisms to go back to its original ground state S
0
. There are
numerous decay processes; the two important types are nonradiative decay, where energy is lost by vibronic motions, or by
radiative decay, in which energy is lost by fluorescence. Fluorescence is an important property that is manipulated in fluo-
rescence microscopy. It can be quantified by monitoring the emission quantum yield and the emission lifetime (decay),
which dictate the selection criteria for designing fluorophores. The fluorescence quantum efficiency of a fluorophore is the
ratio of fluorescence photons emitted to the photons absorbed [31]. It is given as a quantum yield percentage where the
quantum efficiency of emission is calculated using standard samples (which have a fixed and known fluorescence quantum
yield value) according to Equation (1.5) [32]:
Cy5
N
+
N
R
R
I
-
FIgure 1.18
cy5, a typical dye used in optical imaging.
S
1
T
1
S
0
FIgure 1.19
Jablonski diagram showing the basic principles of fluorescence.


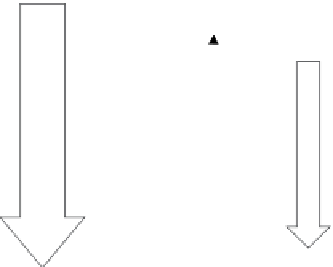







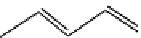

















