Chemistry Reference
In-Depth Information
intrinsic structural and absorption differences in the properties through which the X-rays travel, such as tissues, organs,
bone, fat, water, and air [20].
In cT imaging, cross-sectional images of body organs and tissues are obtained at very high resolution, thus allowing
differentiation among different types of tissue. The images are reconstructed from computers using the Fourier transform, a
mathematical operation that reconstructs the cross-section to form an image of a slice through the body with the focal point
centred at the position of the X-ray beam. This is the most basic image that can be obtained. other more advanced image
reconstruction, such as 3D images, can also be produced by taking multiple scans at short intervals and then stacking the
slices together. Dynamic spatial reconstruction (DSr) is also possible by using approximately 30 X-ray tubes to produce
images that show changes through time; thus, dynamic changes in structure and function can be monitored [20, 21]. cT
imaging can provide views of soft tissue, bone, muscle, and blood vessels without compromising clarity. other imaging
techniques are much more limited in the types of images they can provide, so cT is commonly used for diagnostic purposes
[21]. With the use of contrast agents such as iodinated contrast agents, imaging of tumours is also possible, providing
information on size and localisation, thus aiding planning in treatment, whether for surgery or radiotherapy.
The use of higher energy X-rays can also improve resolution but can be problematic especially in the health of human
patients because it increases ionizing radiation damage. normally, higher X-ray cT machines are used for animal studies.
As mentioned previously, cT images are generated by creating slices through the body; this is recorded as areas of varying
cT intensities and represented in pixels. These pixels are represented by a number that is quantitative to the amount of X-ray
beam absorbed by the tissues at each individual point in the body.
The image is created according to the density of the tissue from a matrix of pixels that are converted to cT numbers
known as Hounsfield numbers (HU, Eq. 1.2). This scale is defined in Hounsfield units (HU) and shown in Figure 1.11.
The denser the tissues are, the higher the value of the numbers, thus a greyscale is created. Different windows and levels
are created from these numbers to produce an image. Different size windows affect the quality of the image by defining
the range as well as the upper and lower limits. For example, a large window shows the major structures, whereas a
small window shows finer details that are often used to discern tissues of similar density. The Hounsfield number at the
centre of the window is referenced at the level and is used to define the range for the window associated with the type
of structure of interest. To enhance cT images, high density contrast agents as well as multi-slice detector geometries
that allow whole-body imaging are used; these help to reduce scanning times and patient discomfort as well as
artefacts.
=
µµ
µ
−
HU
X
ater
×
1000
(1.2)
−
µ
Water
X
Hounsfield scale
3000
Blood
Liver
Tumour
60
Kidneys
Spleen
Heart
Pancreas
Bone
Adrenal
gland
Bladder
40
Intestine
Water
0
-100
Mamma
-200
Fat
-900
Lung
Air
-1000
FIgure 1.11
The Hounsfield Scale and the equation for the Hounsfield Unit (Eq. 1.2).
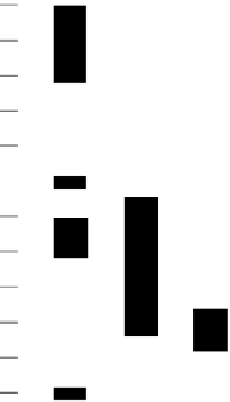
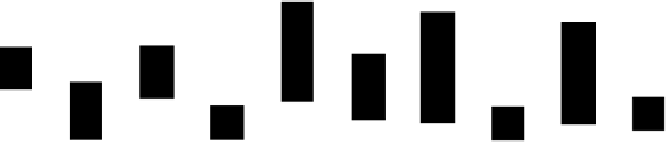


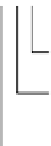




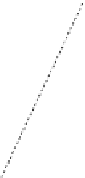




