Biomedical Engineering Reference
In-Depth Information
Each form of regenerated tissue at various
healing stages possesses unique viscoelastic
properties that depend on the maturity of the
collagen network and the mineralization of the
hydroxyapatite frames. In all cases, the tissue
should be strong enough to sustain deforma-
tion but compliant enough to allow deforma-
tion. An exact deformation that is within the
tolerance of the mechanical strength of the
tissue and beyond the threshold of cellular sen-
sitivity is important for the success of regenera-
tive therapies.
is expressed in the dimensions of force per unit
area [
]. These nine components of stress form
a symmetrical mathematical matrix in a carte-
sian coordinate system. Each stress component
is expressed by the orthogonal vectors in the
cartesian system, which represents the view-
point of the observer. The magnitude of each
stress component changes when the system
rotates. At one particular rotation angle, we
can eliminate confounding dimensions, thus
reducing the system to a simpler diagonal
matrix with three nonzero stresses.
The coordinate axes of the diagonal matrix
are called principal axes, and the correspond-
ing stress components are called principal
stresses. In engineering terms, the maximum
of these principal stresses is referred to as the
tensile stress
, and the minimum is referred to
as the
compressive stress.
In physics, tensile
stresses pull the neighboring ECM molecules
apart, which tends to unfold and straighten
protein molecules. Compressive stresses, on
the other hand, condense neighboring parti-
cles, which shortens the distance between
hydroxyapatite crystals in mineralized bone
and affects the growth of the minerals. In addi-
tion, the same cells in a given area will respond
differently to tensile and compressive stress.
When a force is applied to tissue, both of these
stresses combine to alter bone homeostasis at
the local site. Such a differential effect of
stresses becomes critical when motion, such as
distraction, is used to regenerate bone.
Functionally, skeletal regeneration is an
extension of adaptive responses, which are con-
trolled by genetically and environmentally
determined factors. Mechanical adaptation,
paralleling Wolff's law to a great degree, results
in remodeling of the proteins and minerals
within ECM to accommodate the applied stress
patterns. Stresses can be carefully engineered
along a specifi c direction, and thus designing
mechanotherapies where stresses are controlled
may assist in providing predictable regenera-
tive approaches. Two clinical examples using
such strategies are distraction osteogenesis for
lengthening of limbs and jaw bones, and alveo-
lar bone regeneration through control of tooth
movement.
39
8.3 Motion and Osteogenesis
The physical interaction between the various
cells and their resulting ECM must be consid-
ered. In continuum mechanics, motion can
create a relative movement in ECM to a selected
reference point that needs to be considered in
a three-dimensional space. Internal stresses
and strains created by motion are transmitted
in various directions to maintain equilibrium
of cells and associated ECM within the local
environment. From the mechanical theory,
nine components of stresses and strains, as
illustrated in Fig.
8
.
1
, exist at any point in ECM.
The components
σ
zz
are considered
normal stresses, and the remaining compo-
nents
σ
xx
,
σ
yy
,
σ
zy
, etc. are consid-
ered shearing stresses. Each of these components
σ
xy
,
σ
xz
,
σ
yx
,
σ
yz
,
σ
zx
,
σ
yy
σ
yx
σ
xy
σ
yz
σ
xx
σ
zy
σ
xz
σ
zx
σ
y
zz
x
8.3.1 Distraction Osteogenesis
z
Distraction osteogenesis provides an attractive
model for the study of mechanical forces and
Figure 8.1.
Schematic drawing of nine stress components at
any point inside an object.
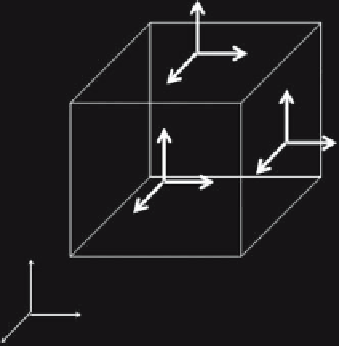