Biomedical Engineering Reference
In-Depth Information
Fig. 2.2. Conceptual representation of dynamic properties of temporal biological sig-
nals and their complexity with reference to ranges of normal and abnormal biological
function. Note that truly random dynamics is the highest degree of complexity which is
not typical to the normal, physiological function, just as well as one that either decays
to zero or exhibits a level with no variations in time. From this latter state, increasing
energy flux takes the system to higher levels of complexity. If the number of deter-
minants is small, the complex dynamics can likely be chaotic, while in most biolog-
ical systems, due to the very large number of simultaneously operating factors, the
dynamics can likely be of a temporal fractal, which shows self-similar pattern of fluc-
tuation as observed across a range of time scales. Physiological dynamics are to be
found within a restricted range of dynamic complexity. Disease states are typically
associated with a disruption of complexity in directions of decreasing and increasing
dynamics.
Our task at revealing the significance of dynamics in phys-
iology and pathology should begin with the understanding of
how complexity in these signals can be quantitatively character-
ized. Two major trends of analysis (
Fig. 2.2
) have emerged in
this respect in the past decades: One (chaos theory) that can
be applied to a deterministic system with a few control param-
eters in action, the other applicable to a system in which the
dynamics result from the simultaneous impact of a large number
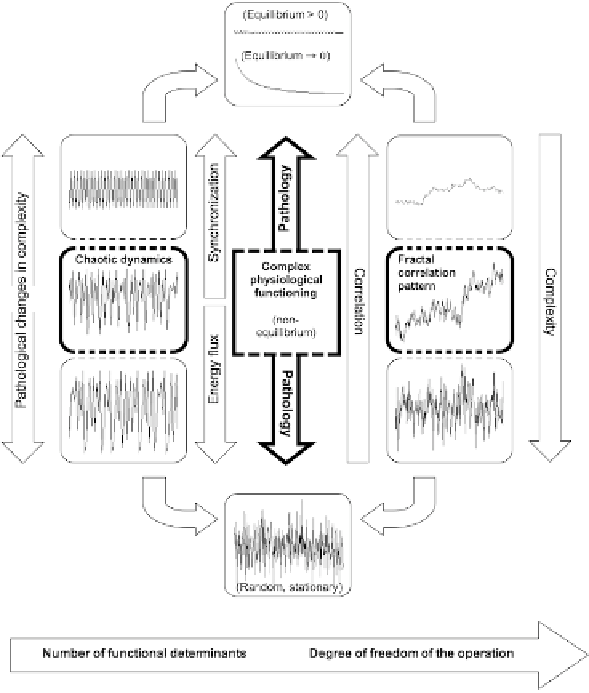