Biomedical Engineering Reference
In-Depth Information
continuum
models
MSM
LDM
0
50
100
150
200
250
300
350
μ
Vessel diameter ( m)
Fig. 10.26.
Applicability of different models with respect to a characteristic vessel size (diameter)
presented accurate predictions of the bulk viscosity both for healthy blood as well
as for infected blood with parasitemia levels up to 100 %.
The two RBC models we presented can be used in a complementary fashion in
simulations of hematologic disorders. The multiscale model (MS-RBC) can resolve
structures down to protein level on the lipid bilayer or the spectrin level of the cy-
toskeleton and can be used efficiently for whole blood simulations (up to 45 % hema-
tocrit) for capillaries and arterioles of approximately up to 100 microns in diameter.
On the other hand, the more economical low-dimensional model (LD-RBC) does not
account for the membrane explicitly and it is only accurate, as we demonstrated in
Sect. 10.4, for arterioles, i.e. vessels with diameter above 15-20 microns. That too,
however, can become computationally expensive for high hematocrit values and for
large arteries. To this end, a continuum model can be employed above a certain ves-
sel size with the Newtonian constitutive law valid for blood for that size of arterial
vessels. This multiscale approach, which is appropriate for the entire human arterial
tree, is demonstrated diagrammatically in the sketch of Fig. 10.26.
Next, we comment further on the RBC model parameters used as input from ex-
perimental data, which can be roughly divided into three groups: (1) RBC properties,
e.g., geometry, shear and bending moduli, membrane viscosity, parasite in malaria,
polymerized hemoglobin in sickle cell anemia, etc.; (2) adhesion properties, e.g.,
receptor and ligand densities, on and off rates, and bond stiffness, and (3) flow prop-
erties, e.g., shear rate, fluid viscosity. The flow properties can be effectively reduced
to a single parameter, e.g., the wall shear stress (WSS), which is the governing pa-
rameter and can influence greatly the adhesive dynamics as we demonstrated in the
case of malaria. Often the dominant RBC parameter is the shear modulus not only in
the static but also in the dynamic response. For example, the increased shear modu-
lus of Pf-RBCs in comparison with healthy RBCs is the main reason for the flipping
motion of Pf-RBCs. Cell geometry could be also very important, for example more
spherical Pf-RBCs will likely roll on a surface than flip; shape changes are particu-
larly influential in sickle cell anemia, spherocytosis and elliptocytosis. On the other
hand, the RBC bending rigidity seems to play a secondary role in the mechanics or
dynamics, and in most cases the membrane viscosity also plays a secondary role, but
not for very small capillaries, as we discussed in Sect. 10.4.1. For diseased RBCs,
the adhesion parameters govern the states of adhesion, e.g., firm adhesion, flipping,
slipping, detachment. For leukocytes, these different states have been studied thor-



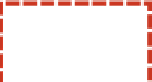




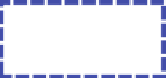















