Biology Reference
In-Depth Information
(A)
(B)
(C)
e
-
e
-
e
-
e
-
e
-
FIGURE 16.4
Combining artificial and biological light-capture mechanisms for energy generation. (A) S. oneidensis cells in a bioreactor expressing proteorhodopsin
(orange). Upon light excitation, proteorhodopsin (PR) generates a proton gradient which can be used by the cell for ATP synthesis or lactate import. Excess
reducing equivalents generated during cell growth are transferred through the S. oneidensis metal reduction pathway (red) via either direct contact or an
intermediate to the electrode surface generating a current. (B) Purified bacterial reaction centers (yellow and green) attached to an electrode. Upon light
excitation, electrons are driven from the RCs to the electrode generating a current. The low-energy electrons at the special pair are regenerated from
synthetic electron donors (red) present in the solution. (C) S. oneidensis cells coupled to an artificial light-harvesting system (blue) either directly through the
metal reduction pathway (red) or through a mediator. The additional energy from the light-energy conversion can be used by the cell during the production of
complex, value-added products (orange).
(
Fig. 16.4
). If
S. oneidensis
is able to interface with such a system, it would eliminate the
need to engineer a biological light-harvesting system into the cell, or the need to drive
electrons into
S. oneidensis
using an electrode, simplifying the final system. A
S.
oneidensis
nanotube interface could be set in a standard bioreactor, or perhaps the cells
could be immobilized in a solid matrix.
313
The reverse scenario is also intriguing. Creating photovoltaic cells which couple isolated core
RC proteins, with a sensitized nanotube or an electrode, has the potential to dramatically
increase the efficiency of the light-capturing reactions, thus boosting the final electrical
output of the photovoltaic cell (
Fig. 16.4
). Efforts to do this are currently underway, and it
had been demonstrated to be a viable system.
54
The studies have also shown that including
other cofactors, such as a cytochromes, increases the efficiency of electron transfer from the
RC to the electrode.
54,55
Ensuring the presence of proper accessory proteins has been a
theme throughout this chapter, and it remains true when working in vitro on an engineered
surface, such as an electrode.
FUTURE DIRECTIONS
There is little doubt about the numerous benefits and potential biotechnological application
that can be realized by combining photosynthetic machinery with the biosynthesis of
industrially relevant compounds. Initial steps in this direction have been taken, though
there are still many hurdles to overcome before a seamless incorporation of light-capturing
complexes in a nonphotosynthetic host is possible. Synthetic biology offers many of the
tools required to successfully integrate light-capture into novel hosts and novel applications.
Applications may include introducing light-energy conversion machinery into a host
natively able to generate electricity, effectively creating biological photovoltaic cells, or
modifying current photovoltaic cells by adding purified light-harvesting components to
increase light-capture efficiency. Another exciting possibility is combining artificial light-
capture mechanisms, like photosensitized nanotubes, with a biological system producing
value-added products. However, some hurdles like inability to express BchE recombinantly,
or optimizing the interface between a biological and artificial system, still remain. The
combination of biology and engineering allows for the development of creative solutions to
overcome current hurdles which neither field alone could overcome.

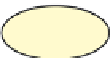

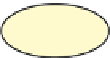




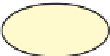




















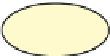










































































