Biology Reference
In-Depth Information
We mentioned previously that organisms such as
S. oneidensis
and
G. sulfurreducens
are able
to balance any excess-reducing equivalents by shuttling those electrons onto an electrode,
thus generating a current, and it has been shown that the same process can be driven in
reverse.
48
Driving electrons back into
S. oneidensis
through the Mtr pathway generates
reduced quinones that can
be oxidized to regenerate energy carrying molecules
such as NAD(P)H. While this system needs further testing, it is an attractive prospect to
engineer a bacterium such as
S. oneidensis
to fix CO
2
and produce high value-added
commodity chemicals by using electricity as an energy source for the cell.
'
in theory
'
FROM BIOLOGICAL TO ARTIFICIAL PHOTOSYNTHETIC SYSTEMS
Nature has had millions of years to perfect photosynthetic machinery; however, for specific
applications, a smaller, artificial mimic might be more desirable. For example, it is difficult
to utilize a photosynthetic organism in a photovoltaic cell, or conditions in a particular
application may be too harsh, damaging the cells. In such instances, a chemically
synthesized mimic of a naturally occurring process may offer a solution. The robust nature
and functionality of photosynthesis is a marvel of biological mechanics, and a great deal of
research has focused on artificially replicating different parts of the photosynthetic process
(reviewed in
49,50
). Investigations have yielded new catalysts such as TiO
2
that are able to
split water into hydrogen and oxygen when excited by the UV light.
51
Synthetic compounds
have also been developed that mimic chlorophylls and are used to transfer electrons to a
conductive surface once they have been excited by light (
Fig. 16.3
). A majority of the dyes
utilized in engineered photosynthetic systems are derived from a chemically modified
porphyrin backbone, which has an absorption wavelength in the 400 nm range.
50
Porphyrin-derived dyes are excited by light and donate a high-energy electron, similar to
what the special pair can do in the bacterial RC. Once the electron is donated from a
synthetic dye, it is replaced by an electron from an electrolyte solution, creating a noncyclic
system unlike that of the type II RCs where the electron must return to its source.
312
Recent research into artificial photosynthesis has attempted to couple light excitation to the
reduction of NAD(P)
1
, essentially mimicking what occurs during photosynthesis in
nature.
52,53
These techniques involve photosensitizing a nanotube made from a conductive
material such as TiO
2
or diphenylalanine (F-F). Photosensitizing compounds can be
artificial, such as quantum dots, or of biological origin, such as porphyrin mimics.
52
A
mediator compound is used to transfer the electrons from the nanotubes onto NAD(P)
1
molecules. This is exciting research, as it would allow for the regeneration of reducing
equivalents in vitro, using only sunlight, replacing the multienzyme systems currently used.
The next step could be combining an artificial light-harvesting nanotube system with a
bacterium such as
S. oneidensis
, which is able to utilize external electrons for biosynthesis
FIGURE 16.3
Examples of photoactive dyes typically used for artificially engineered systems. (A) A dinuclear, Mn-based water-oxidation catalyst that mimics the water
splitting by the oxygen-evolving photosystem II. (B) A biological mimic dye containing elements from a carotenoid, porphyrin, and quinone molecules joined
together. (C) An example of a Ru-based dye used in dye-sensitized solar cells.
49,50


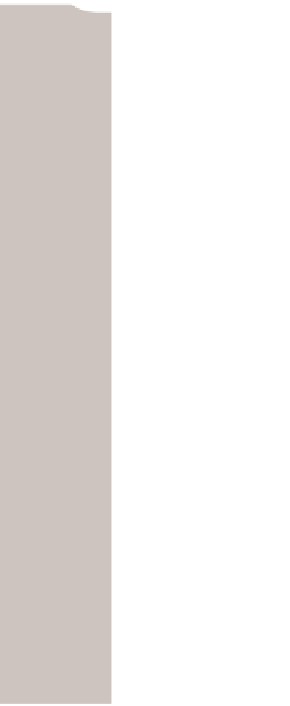