Biology Reference
In-Depth Information
s
27
so-called DDMI
Another general approach for steps 1 and 2 is represented by Jha et al.
'
method (
'
Docking, sequence Design, and gradient-based Minimization
'
). In this method,
first protein
protein docking methods are used to place a candidate scaffold (taken from a
library) and the desired target in close proximity to each other. During the docking phase,
the energy function is augmented with restraints that direct the scaffold protein towards the
desired binding site on the surface of the target protein. At the end of the docking phase, a
rough score filter is used to determine whether the candidate complex will be discarded or
subjected to the next sequence design phase of the protocol. During this phase, the docked
complex model is then subjected to iterative rounds of sequence design and gradient-based
minimization, and resulting sequences are selected for expression based on a combination
of energy function scores and structural parameters. The authors used their method to
generate six designs against PAK1, a human kinase, the best of which bound with an affinity
of 100
μ
M to the desired target.
Several other approaches for steps 1 and 2 that rely on the target protein to have certain
structural features exist. While none of them are as general as the hotspot design or DDMI
approach, they have been shown to yield high-affinity binders against targets having the
required features. Two cases of exploiting solvent-exposed edges of beta-sheets to form a
binding site have been reported. This strategy makes use of the fact that such edges have
accessible unsatisfied hydrogen-bonding functionalities, which can be bound relatively
easily by any scaffold that also has a beta-sheet with an exposed edge, thus forming an
intermolecular beta-sheet upon complex formation. The residues surrounding this beta-
sheet anchored interface are then redesigned to further increase the affinity and modulate
specificity beyond that provided by the sheet-contacts alone. Stranges et al.
28
used this
approach to turn a monomeric protein into a symmetric homodimer. Starting from a library
of proteins with exposed beta-strands, the authors generated initial models consisting of
two copies of the monomer interacting through the strand, followed by, like in most design
protocols, cycles of sequence design and minimization. Four designs were experimentally
characterized, the best of which had a K
D
of 1
109
M, and a crystal structure showed that the
interface was virtually super-imposable onto the design model. Sievers et al. used a very
similar approach to design peptides that inhibit amyloid formation.
2
μ
The strong interactions that some amino acids can make with metals can also be harnessed
to design protein interfaces. The same way in which a metal site can significantly stabilize a
protein structure can also be used to stabilize an interface. The coordination spheres of
metals usually contain between four and six ligand sites. Thus, if a site containing half of a
metal coordination sphere can be designed on the surface of a protein, two copies of this
protein could then dimerize and form the complete metal site. Der et al.
29
demonstrated
the feasibility of this approach by designing a zinc-mediated homodimer from a monomeric
scaffold protein. The design had a very tight interface (K
D
,
30 nM) in the presence of zinc,
but in the absence thereof, the K
D
increased
100-fold, indicating the importance of the
metal. Interestingly, as demonstrated by Salgado et al.,
30
this approach can also be used as a
stepping stone to design interfaces not dependent on metal. In their example, the authors
first created a minimalist interface featuring a zinc-binding site, and then used CPD to create
additional interactions across the interface, resulting in a design where binding was
independent of metal presence.
B
Finally, another viable strategy to create novel protein binders against a given target is the
so-called grafting of functional epitopes. This method relies on the availability of an already
existing binder for the target of interest, and preferentially a crystal structure of the complex
between the two. In this approach, the binding residues of the existing binder are
transferred onto another scaffold, thus endowing this scaffold with binding affinity for the
target of interest. This method is useful in cases where, for example, the original scaffold is
difficult to express or problematic in other ways. Further, as demonstrated by Sia et al.,
31

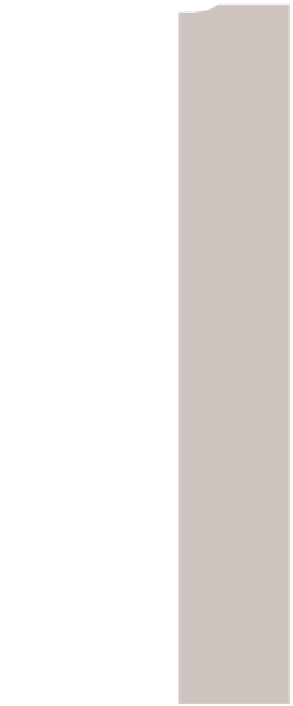