Geoscience Reference
In-Depth Information
below about 10 or 15 km and as the maximum possible
water vapour density of cold air is very low anyway (see
B.2, this chapter), there is little water vapour in the upper
layers of the atmosphere.
Ozone (O
3
) is concentrated mainly between 15 and
35 km. The upper layers of the atmosphere are irradiated
by ultraviolet radiation from the sun (see C.1, this
chapter), which causes the breakup of oxygen molecules
at altitudes above 30 km (i.e. O
2
→ O
O). These
separated atoms (O + O) may then combine individu-
ally with other oxygen molecules to create ozone, as
illustrated by the simple photochemical scheme:
O
2
O
M
→
O
3
M
where M represents the energy and momentum balance
provided by collision with a third atom or molecule;
this Chapman cycle is shown schematically in Figure
2.2A. Such three-body collisions are rare at 80 to
100 km because of the very low density of the atmos-
phere, while below about 35 km most of the incoming
ultraviolet radiation has already been absorbed at higher
levels. Therefore ozone is formed mainly between
30 and 60 km, where collisions between O and O
2
are
more likely. Ozone itself is unstable; its abundance
is determined by three different photochemical
interactions. Above 40 km odd oxygen is destroyed
primarily by a cycle involving molecular oxygen;
between 20 and 40 km NO
x
cycles are dominant; while
below 20 km a hydrogen-oxygen radical (HO
2
) is
responsible. Additional important cycles involve
chlorine (ClO) and bromine (BrO) chains at various
altitudes. Collisions with monatomic oxygen may re-
create oxygen (see Figure 2.2B), but ozone is destroyed
mainly through cycles involving catalytic reactions,
some of which are photochemical associated with
longer wavelength ultraviolet radiation (2.3 to 2.9 µm).
The destruction of ozone involves a recombination
with atomic oxygen, causing a net loss of the odd
oxygen. This takes place through the catalytic effect of
a radical such as OH (hydroxyl):
Figure 2.2
Schematic illustrations of (A) the Chapman cycle of
ozone formation and (B) ozone destruction. X is any ozone-
destroying species (e.g. H, OH, NO, CR, Br).
Source
: After Hales (1996), from
Bulletin of the American Meteorological
Society
, by permission of the American Meteorological Society.
Stratospheric ozone is similarly destroyed in the
presence of nitrogen oxides (NO
x
, i.e. NO
2
and NO) and
chlorine radicals (Cl, ClO). The source gas of the NO
x
is nitrous oxide (N
2
O), which is produced by com-
bustion and fertilizer use, while chlorofluorocarbons
(CFCs), manufactured for 'freon', give rise to the
chlorines. These source gases are transported up to
the stratosphere from the surface and are converted by
oxidation into NO
x
, and by UV photodecomposition
into chlorine radicals, respectively.
The chlorine chain involves:
2 (Cl
O
3
→ ClO
O
2
)
ClO
ClO → Cl
2
O
2
and
Cl
O
3
→
ClO
O
2
OH
O
3
→
HO
3
2O
2
Both reactions result in a conversion of O
3
to O
2
and
the removal of all odd oxygens. Another cycle may
involve an interaction of the oxides of chlorine and
bromine (Br). It appears that the increases of Cl and Br
species during the years 1970 to 1990 are sufficient
to explain the observed decrease of stratospheric ozone
over Antarctica (see Box 2.1). A mechanism that may
enhance the catalytic process involves polar strato-
spheric clouds. These can form readily during the austral
H
HO
2
HO
2
O → OH
O
2
O
→
net: 2O → O
2
OH
O → H
O
2
The odd hydrogen atoms and OH result from the dis-
sociation of water vapour, molecular hydrogen and
methane (CH
4
).
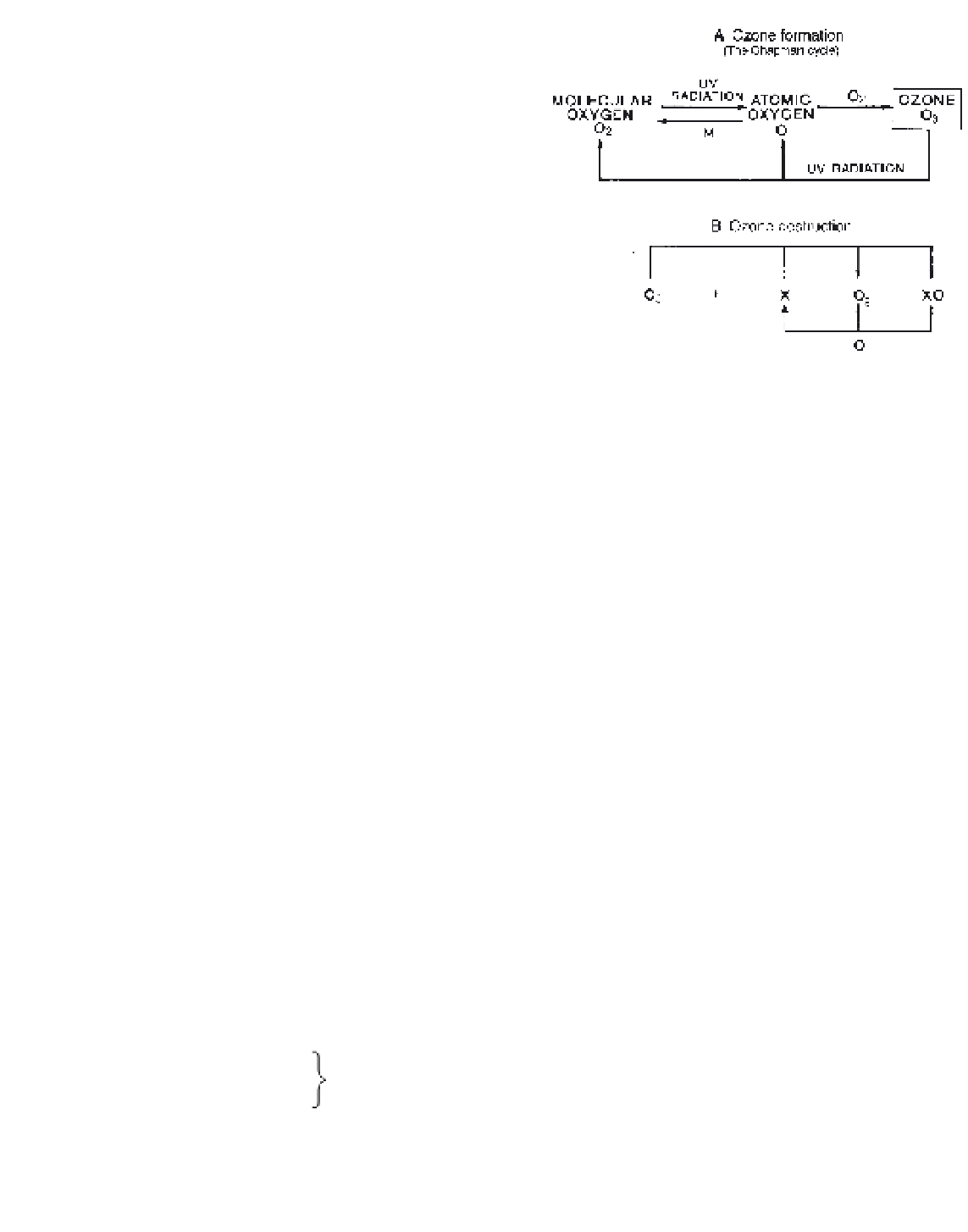