Geoscience Reference
In-Depth Information
photochemical origin associated with anthro-
pogenic activities accounts for about half of these
in the Northern Hemisphere. Dimethyl sulfide
(DMS), associated with algal decomposition,
also undergoes oxidation to sulfate. Over the
tropical continents, aerosols are produced by
forest vegetation and surface litter, and through
biomass burning; particulate organic carbon
predominates. In mid-latitudes, remote from
anthropogenic sources, coarse particles are mostly
of crustal origin (calcium, iron, potassium and
aluminium) whereas crustal, organic and sulfate
particles are almost equally represented in the fine
aerosol load.
Aerosols have a substantial effect on cloud
properties and therefore on the initiation of
precipitation. Polluted atmospheres typically have
aerosol concentrations x 10-100 times those of
pristine oceanic air masses. The effects of aerosols
on clouds involve radiative processes and
microphysical effects. Aerosol layers decrease
the solar radiation reaching the surface acting to
lower surface temperatures and reduce evapora-
tion and convection. Aerosols also serve as cloud
condensation nuclei (CCN), which by nucleating
large numbers of small droplets, slow the
conversion of cloud droplets into raindrops. The
net effect seems to be to decrease precipitation
from shallow clouds, but to enhance precipitation
from deep convective clouds with warm cloud
bases. The maximum enhancement occurs for
intermediate CCN values, whereas at higher
concentrations both the radiative and micro-
physical effects work towards a lower release of
convective energy.
Hygroscopic aerosols are soluble. This is very
important since the saturation vapor pressure is
less over a solution droplet (for example, sodium
chloride or sulfuric acid) than over a pure water
drop of the same size and temperature (
Figure
5.8
). Indeed, condensation begins on hygroscopic
particles before the air is saturated; in the case of
sodium chloride nuclei at 78 percent relative
humidity.
Figure 5.8
illustrates Kohler curves
showing droplet radii for three sets of solution
0.3
0.2
Pure water
10
-16
g
0.1
10
-15
g
10
-14
g
100
NaCl solution
droplets
90
80
10
-6
10
-5
10
-4
10
-3
Droplet radius (cm)
Figure 5.8
Kohler curves showing the variation
of equilibrium relative humidity or supersaturation
(%) with droplet radius for pure water and NaCl
solution droplets. The numbers show the mass
of sodium chloride (a similar family of curves is
obtained for sulfate solutions). The pure water
droplet line illustrates the curvature effect.
droplets of sodium chloride (a common sea salt)
in relation to their equilibrium relative humidity.
Droplets in an environment where values are
below/above the appropriate curve will evaporate/
grow. Each curve has a maximum beyond which
the droplet can grow in air with less super-
saturation.
Once formed, the growth of water droplets is
far from simple. In the early stages, the solution
effect is predominant and small drops grow
more quickly than large ones, but as the size of a
droplet increases, its growth rate by condensation
decreases (
Figure 5.9
). Radial growth rate slows
down as the drop size increases, because there is a
greater surface area to cover with every increment
of radius. However, the condensation rate is
limited by the speed with which the released latent
heat can be lost from the drop by conduction to
the air; this heat reduces the vapor gradient. In
addition, competition between droplets for the
available moisture acts to reduce the degree of
supersaturation.

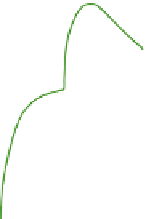

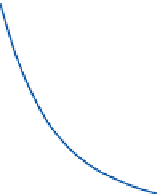
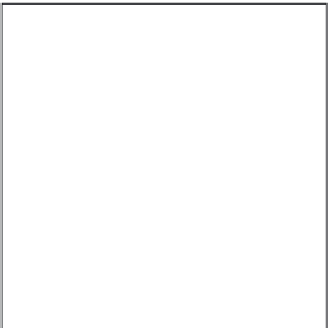




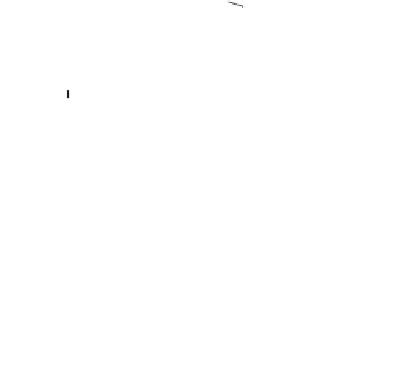









