Geoscience Reference
In-Depth Information
Figure 2.8. The buoyancy distribution from (2.72) (left panel) and the perturbation pressure
field associated with it (right panel) (from Parsons and Kropfli, 1990), but with negative
buoyancy, rather than positive buoyancy. Note how there is an upward-directed perturbation
pressure gradient force ( p
0
<
0 above and p
0
>
0 below) that acts opposite to the imposed
negative buoyancy.
at using Archimedean buoyancy as a force. Finally, for the case when H
¼
L,itis
seen that the vertical acceleration due to buoyancy is cut down to one half of what
it would have been in the absence of the opposing vertical perturbation pressure
gradient force. The reader is reminded that this simplified analysis does not include
the effects of turbulent entrainment of environmental air into the bubble and the
effects of phase change of water substance. In addition, this analysis does not
include the effects of the interaction of the updraft in the bubble with the environ-
mental wind field, which so far has been assumed to be calm. The latter (via p
0
d
)
will be considered later when we analyze downdrafts, microbursts, and supercell
convection.
2.5.2 Retrieval of pressure and buoyancy fields from the wind field
To retrieve the thermodynamic profiles from wind observations (particularly those
synthesized from Doppler radar wind data), one can use a technique pioneered by
Tzvi Gal-Chen in the early 1980s at the University of Oklahoma, which is based
on a divergence equation for the Boussinesq system of equations, as follows:
the perturbation pressure field at each level is solved from the horizontal (two-
dimensional) version of the divergence equation (2.62); the buoyancy field does
not appear in the horizontal divergence equation
h
p
0
¼
1
=
½@=@
x
ð
Du
=
Dt
Þþ@=@
y
ð
D
v=
Dt
Þ
ð
2
:
76
Þ
J
Since time derivatives appear, it is necessary that the Doppler radars sample the
atmospheric wind field frequently enough to minimize aliasing of high-frequency
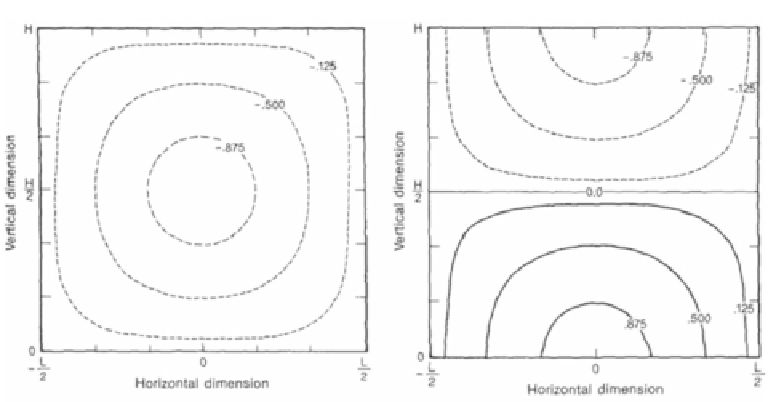
Search WWH ::

Custom Search