Geoscience Reference
In-Depth Information
the processes and their responses are shown again by
positive and negative controls. An increase in slope erosion
will lead to an increase in stream bed load, which in turn
causes a decrease in channel erosion, and eventually a
decrease in valley-side slope angle, and ultimately a
decrease in slope erosion.
The 'knock-on' effects of change within any process-
response system take the form of
feedback
loops. Feedback
refers to 'the feeding back of part of the output of a system
as input for another phase of operation, especially for self-
correcting or control purposes' (Chorley and Kennedy
1971).
Negative feedback
, like the slope erosion example
above, acts in a conservative manner; a change in the
cascade produces morphological changes which create
variations in the cascading system, leading to a damping
down of the effects of the original change. In short,
negative feedback slows down the rate of change.
Positive
feedback
occurs when closed loops reinforce the effects of
change to give 'snowballing' of the changes in the same
direction as their initial action. In short, positive feedback
speeds up the rate of change.
Examples of negative and positive feedback abound in
any environmental system. The climate system, with its
many complex effects of feedback, that will determine
future climate scenarios under global warming, is a prime
case. A climate example of negative feedback is the
water
vapour effect:
global warming leads to more water vapour,
and hence thicker cloud cover, and therefore more
reflection of solar radiation, thus slowing down the rate
of climatic change. An example of positive feedback is the
ice albedo effect:
global warming leads to ice melting,
a lower albedo, faster radiation absorption, higher air
temperatures and hence more melting of ice.
normal science
. Knowledge and understanding advance in
any academic field largely through the efforts of revolu-
tionaries who are able to move outside the prevailing
paradigm, challenge accepted views and successfully
develop a new paradigm, thus producing a
paradigm shift
.
Kennedy (2006) notes that two paradigms rarely coexist,
and
scientific revolutions
are born when a new paradigm
vies for supremacy over a traditionally accepted one.
However, every paradigm leaves its legacy on how a
discipline is taught and researched, and past paradigms
are rarely wiped out completely.
Throughout its history, physical geography has
undergone several revolutions in either concepts and/or
methodologies. It has progressed far as an academic
discipline in the past two centuries. In 1800 the opinion
of James Ussher, a seventeenth-century Archbishop of
Armagh, that the Earth was created in 4004 BC, still held
sway. What processes, he and subsequent scientists asked,
other than the catastrophe of the biblical Flood could have
fashioned the Earth's surface within the short span of
6,000 years? The alternative possibility of gradual change
over a much longer time period (i.e.
gradualism
rather
than
catastrophism
) was born in the Scottish Enlighten-
ment of the eighteenth century. James Hutton (1726-97)
observed the slow rates of erosion and sedimentation
in the Scotland of his day, and was convinced that the time
since the moment of Creation was effectively indefinite.
Further development of the Huttonian paradigm came
from John Playfair (1747-1819), who initiated the
study of fluvial geomorphology, from Charles Lyell
(1797-1875), who championed the use of scientific
principles and field observations, and from Archibald
Geikie (1835-1924) whose
Principles of Geology
ran to
nine editions (see also p. 560).
Geomorphology, probably always the premier branch
of physical geography, witnessed three major 'paradigm
shifts' in the nineteenth century. Louis Agassiz (1807-73)
became convinced in the 1830s that the landscapes he was
studying in the French Alps and Switzerland had resulted
from the work of ice sheets and valley glaciers. G. K.
Gilbert (1843-1918) was remarkable for combining
the approaches of experimental and historical science
in his landform studies in the American mid-west. His
1879
Report on the Geology of the Henry Mountains
was
the first of many seminal studies for the US Geological
Survey. His investigations combined the formulation of
laws and concepts, such as the
law of divides
, the
law of
uniform slopes
,
weathering-limited slopes
,
transport-limited
slopes,
with a complementary appreciation that land-
scape is something which has to evolve over extended
time periods. He receives fulsome praise from Barbara
Like all physical landscapes in the real world, Upper
Wharfedale is complex, and becomes more complex the
more the physical geographer explores the detail of its
components and processes. The physical geographer
searches for the truths behind the principles and processes
that govern climates, landforms, oceans, soils and eco-
systems, and is no different from any other natural
scientist seeking to unravel the complexity of the natural
world. The physical geographer also has to work with the
accepted principles of scientific knowledge and belief
prevailing in his discipline at the time, i.e. what Kuhn
(1962) calls the prevailing
paradigm
within a period of
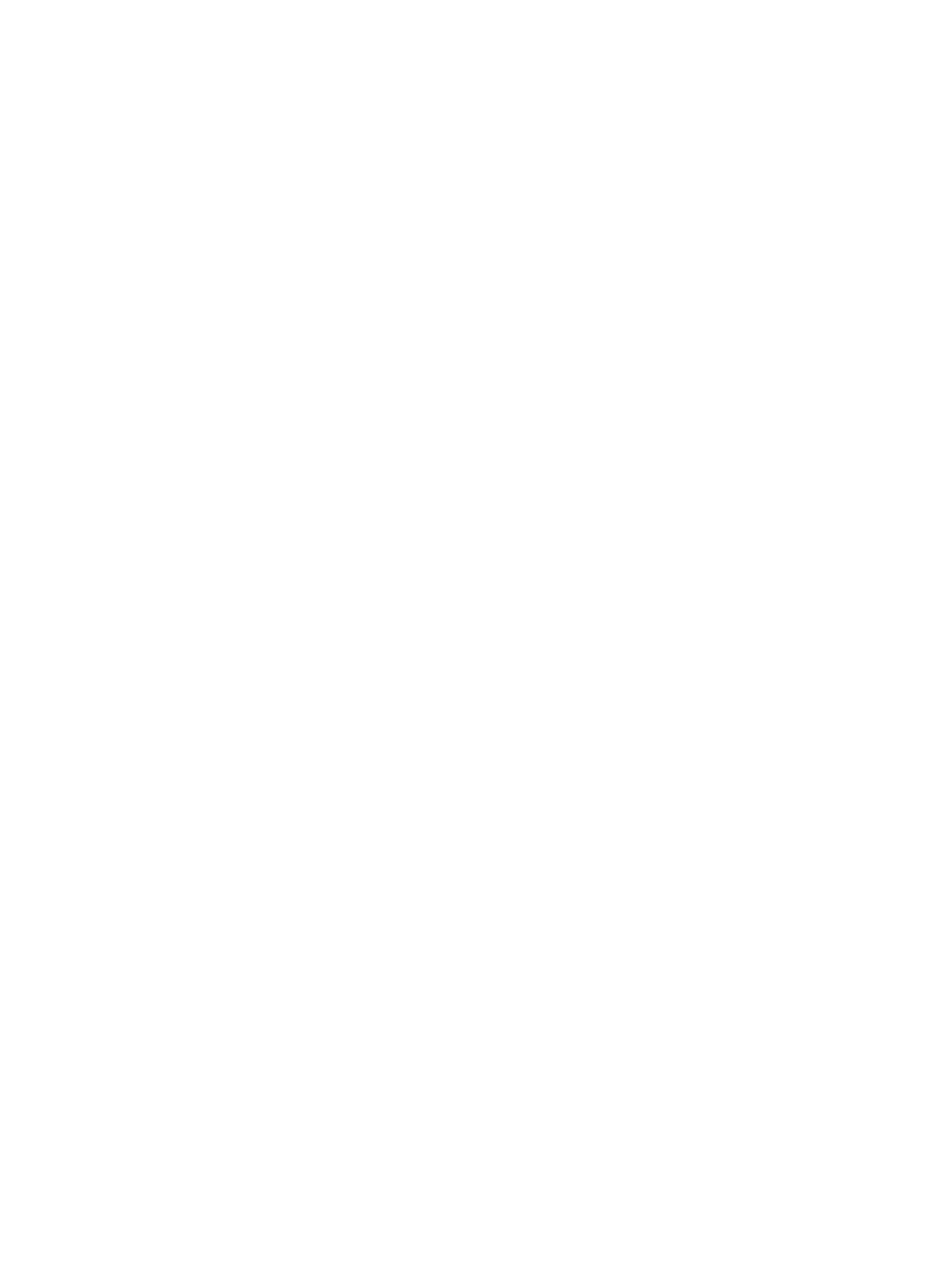






































