Geoscience Reference
In-Depth Information
Nighttime MSTID observations (TEC, Airglow)
OI 630.0 nm emission
270 km
OI 630.0 nm emission
270 km
23:20:00
05/22 '98
Tec [10
14
/m
2
]
46
46
46
0.5
44
44
44
0.0
42
42
42
40
2
0.5
40
40
128
28
130
130
132
132
134
134
136
136
138
138
140
140
142
142
144
144
146
146
14
148
128
146
130
144
132
134
136
140
142
38
138
38
38
1.0
2
36
36
36
1.5
2
34
34
34
32
32
32
30
30
30
(a)
(b)
Figure 6.28b
Simultaneous airglow and TEC observations over Japan. Each data point
at the left corresponds to one of some 1000 GPS stations. [After Saito et al. (2001).
Reproduced with permission of the American Geophysical Union.] See Color Plate 18.
the north, the images are nearly identical. The implication is that the electrifica-
tion of the structures is dominant, since it is very unlikely that identical neutral
gravity waves would occur in both hemispheres.
6.6.3 Linear Theory for the Perkins Instability
By the late 1970s, Behnke (1979) had already suggested that these height-layer
bands were due to the Perkins (1973) instability. Before studying any instability,
it is important to first describe the equilibrium for which stability is addressed.
First, we note that without an eastward electric field, equilibrium can only exist
exactly at the magnetic equator where the
J
g
×
B
force due to gravitational cur-
rents can balance the vertical gravity term in the force balance equation:
J
g
×
B
+
ρ
g
=
0
At the equator,
J
g
has magnitude
B
and is directed toward the east. The
Rayleigh-Taylor theory given in Chapter 4 shows that this equilibrium is not
stable. In general, using (2.36b) for
m
ρ
g
/
B
2
M
,
J
g
=
(ρ/
)
g
×
B
, and if the magnetic
field has a dip angle
I
, then the
J
g
×
B
force is
J
g
×
B
=+
ρ
g
cos
I
ˆ
a
z
(6.17)
where the coordinates are defined in Fig. 5.15. This force cannot balance the
gravitational force
ρ
g
=−
ρ
g
a
z
except at the equator, and no stability exists
ˆ
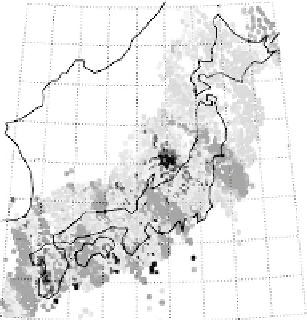

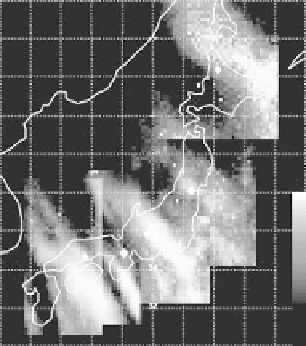
Search WWH ::

Custom Search