Geoscience Reference
In-Depth Information
180
160
140
120
180
160
140
120
P
o
/10
4
NO
1
H
c
/10
2
O
1
2
O
1
Total
100
100
80
80
10
9
10
10
10
11
10
12
10
26
10
25
10
24
10
23
Plasma density (m
23
)
Conductivity (mhos meter
21
)
Figure 3.15
Vertical profiles of daytime composition and plasma density (left) and
conductivities (right) for average solar conditions. [After Forbes and Lindzen (1976).
Reproduced with permission of Pergamon Press.]
5
0
E
z
a
^
<
2
2
2222222222222
2
2 222
E
x
a
^
fi
0
B
H
E
x
P
E
z
J
X
5
P
E
X
1
H
E
Z
<
1
1
111111111111111 111
5
0
J
Z
52
H
E
X
1
P
E
Z
5
0
<
Figure 3.16
The equatorial electrojet in a slab geometry.
eastward along the dayside equator. More importantly, a vertically downward
Hall current will also flow as shown in Fig. 3.16. Now from
∇·
E
=
0, we can
deduce that
∂
/∂
z
=
∂
E
z
/∂
x
E
x
This means that the variation of the zonal component with altitude can be esti-
mated by the ratio
δ
E
x
=
δ
E
z
(δ
z
/δ
x
)
The scale size
(δ
x
)
of the horizontal conductivity pattern is 100 times that
of the vertical
variations of conductivity, whereas both experiments and
theory indicate
E
z
is at most 10-20 times
E
x
. Taken together, this means that the
zonal electric field can change only slightly in the E region. The data presented
in Fig. 3.17b verify this argument, and we will thus assume that conductivity
gradients rather than variations in
E
x
dominate the divergence of the vertical
Hall current. Figure 3.15 shows that the Hall conductivity dominates below
120 km and, indeed, is highly altitude dependent (i.e., a slablike geometry is
appropriate).
As a first approximation to the physics of the E-region dynamo, we again
consider a slab conductivity geometry such as that illustrated in Fig. 3.16, subject
to a constant zonal electric field. The Hall current cannot flow across the bound-
ary, and charge layers must build up, generating an upward-directed electric
(δ
z
)
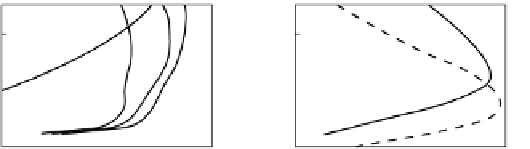

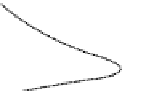















































Search WWH ::

Custom Search