Geoscience Reference
In-Depth Information
energy per unit time, or power (W), absorbed by the
Earth in the simple energy balance model is
The equilibrium temperature of the Earth, 255 K (Exam-
ple 12.1), is 18 K below the freezing temperature of
water and would not support most life on Earth. For-
tunately, the actual globally averaged surface air tem-
perature is about 288 K. The 33 K difference between
the predicted equilibrium temperature and the actual
temperature of the Earth results from the fact that the
Earth has an atmosphere that is transparent to most
incoming solar radiation but selectively absorbs many
wavelengths of outgoing thermal-IR radiation. Some of
the thermal-IR radiation absorbed by the atmosphere is
reemitted to the surface, warming it. The resulting 33 K
increase in temperature over the equilibrium tempera-
ture of the Earth is the
natural greenhouse effect
.
Table 12.2 compares equilibrium temperatures cal-
culated from Equation 12.6 with actual temperatures
of several planets. The planet with the largest differ-
ence between its actual and equilibrium temperatures
is Venus (Figure 12.3a). Venus's surface temperature is
more than 470 K warmer than is its equilibrium temper-
ature. Because Venus is closer to the sun than is the Earth
(Figure 12.3b), Venus receives more solar radiation than
does the Earth, so Venus's temperature, early in its evo-
lution, was higher than was that of the Earth. As a result,
liquid water and ice on the surface of Venus, if ever
present, evaporated and sublimated, respectively. The
resulting water vapor, exposed to intense far-solar UV
radiation, photolyzed to atomic hydrogen [H(g)] and the
hydroxyl radical [OH(g)]. Over time, atomic hydrogen
escaped Venus's gravitational field to space, depleting
the atmosphere of its ability to reform water vapor.
Because the surface of Venus lost all liquid water to
vapor, there was no mechanism to dissolve and convert
its atmospheric CO
2
(g), which built up due to volcanic
outgassing, back to carbonate rock. As its mixing ratio
increased, CO
2
(g) absorbed more thermal-IR radiation,
heating the atmosphere and preventing both condensa-
tion of water and additional removal of CO
2
(g). The
nearly endless positive feedback cycle that occurred on
Venus is called a
runaway greenhouse effect
. Today,
Venus has a surface air pressure ninety times that of the
Earth, and its major atmospheric constituent is CO
2
(g).
Table 12.2 shows that Mercury, Mars, Pluto, and the
moon all have thin atmospheres and little greenhouse
effect. Their atmospheres are thin because light gases
have escaped their weak gravitational fields. Although
Mars's surface pressure is less than 1 percent of the
Earth's, Mars's CO
2
(g) partial pressure is about twenty
times that of the Earth. Because CO
2
(g) is relatively
heavy, it has not entirely escaped Mars's atmosphere,
and because Mars has no oceans, CO
2
(g) cannot be
A
e
)
R
e
P
in
=
F
s
(1
−
(12.4)
This incoming radiation must be equated with radi-
ation emitted by the Earth to derive an equilibrium
temperature.
12.1.2. Outgoing Thermal-Infrared Radiation
The Earth emits radiation at all points on its surface,
which can roughly be represented as a sphere. The
Stefan-Boltzmann law (Equation 2.2) gives the energy
flux emitted by any object, including the Earth. Apply-
ing this law and multiplying through by the surface area
of the Earth, 4
R
e
2
(m
2
), gives the power (W) emitted
by the Earth as
P
out
=
ε
e
B
T
e
4
R
e
(12.5)
where
ε
e
is the globally averaged thermal-IR emissiv-
ity of the Earth (dimensionless) and
T
e
is the
equilib-
rium temperature
(K) of the Earth's surface, which
is the temperature of the Earth without considering the
greenhouse effect. Table 12.1 gives thermal-IR emissiv-
ities for different surfaces. The actual globally averaged
emissivity of the Earth is about 0.9 to 0.98; however,
for the basic calculation presented here, it is assumed
to equal 1.0.
12.1.3. Equilibrium Temperature of the Earth
Equating the net incoming solar power from Equation
12.4 with the outgoing thermal-IR power from Equation
12.5 and solving for the
equilibrium temperature
of
the Earth's surface in the absence of an atmosphere
gives
F
s
(1
1
/
4
−
A
e
)
T
e
=
(12.6)
4
ε
e
B
Example 12.1
Estimate the equilibrium temperature of the
Earth given
F
s
=
1,365 W m
−2
,
A
e
=
0.3, and
ε
e
=
1.0.
Solution
FromEquation 12.6, the equilibrium temperature
of the Earth is
T
e
=
254.8 K, which would be the
temperature of the Earth in the absence of an
atmosphere.
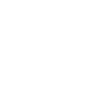
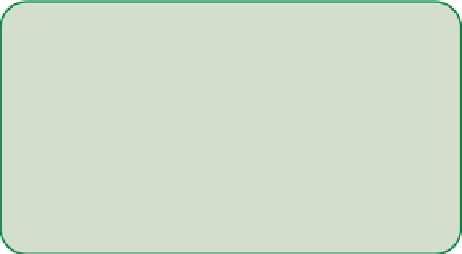
Search WWH ::

Custom Search