Geoscience Reference
In-Depth Information
gives this class a very large density range.
Density (g/cm
3
)
1
.5
2.0
2.5
3.0
0.0
The presence of iron oxides in iron formations makes
these one of the densest rock types.
2.7
2.8 g/cm
3
Coals are amongst the lowest density rocks.
Heavy mineral sands are not particularly dense, because
the effects of the
1.0
components are countered by
high porosities. Even with 50% titanomagnetite content,
only about 2.8 g/cm
3
'
heavy
'
for samples from New Zealand
2.0
A
B
deposits.
Density/compaction curves
r
bulk
= (1
-
f
)
r
matrix
+
f r
pore contents
3.8.4
Changes in density due to metamorphism
and alteration
3.0
= e
-cz
f
f
0
A
f
= 0.65
r
matrix
0
= 2.70 g/cm
3
c
= 0.70
Any alteration or metamorphic reaction involving the
replacement of mineral species with others of different
density will affect the density of a rock. Metamorphic
rocks generally have higher density than the rocks
from which they are derived because the process of
metamorphism reforms the source rocks into more com-
pact forms. Any changes in porosity will also change
its density.
Serpentinisation is arguably the most important alter-
ation/metamorphic process in terms of geophysical
responses, signi
cantly affecting density, magnetism and
acoustic properties. Put simply, serpentinisation is the low-
temperature hydrothermal alteration of olivine and ortho-
pyroxene to serpentine group minerals, brucite and mag-
netite. A comprehensive list of serpentinisation reactions,
in the context of their physical property implications, is
provided by Toft et al.(
1990
).
Figure 3.34
shows that bulk density is inversely
proportional to the degree of serpentinisation. Any rock
containing the requisite minerals may be affected by ser-
pentinisation, but it is especially common in ultrama
4.0
B
= 0.40
= 2.80 g/cm
3
c
= 0.70
f
0
r
matrix
5.0
Figure 3.33
Increase in density with depth in sandstones and
siltstones owing to decrease in porosity associated with compaction.
Redrawn, with permission, from Schön (
1996
).
proportions of low-density felsic to high-density ma
c
minerals, e.g. feldspars and quartz versus amphiboles,
pyroxenes and olivine. Igneous ultramafic rocks continue
this trend, but metamorphic examples tend to be denser,
largely owing to the presence of garnet-bearing eclogites.
Felsic rocks exhibit smaller ranges in density than mafic
and ultramafic rocks. This is due to the susceptibility of
the latter two to alteration, especially serpentinisation
and weathering (see
Sections 3.8.4
and
3.8.5
)
. Also ves-
icles and amygdales may reduce their bulk density.
c
species comprising predominantly serpentinisable min-
erals, e.g. peridotite, dunite, harzburgite and lherzolite.
Ma
c minerals with densities of around 3.1 to 3.6 g/cm
3
are replaced by serpentine-group minerals whose densities
range from 2.55 to 2.61 g/cm
3
. In addition, brucite also has
a low density (2.39 g/cm
3
), and highly serpentinised rocks
may have porosities as high as 15%. Even allowing for the
creation of magnetite (density about 5.2 g/cm
3
), the reduc-
tion in density caused by these effects is signi
cant, with
bulk density decreasing by up to about 0.9 g/cm
3
demon-
serpentinisation is common in mafic and ultramafic rocks
Figures 3.29
and
3.30
show that the density of sedimentary
rocks extends to values that are signi
cantly less than those
of the rock-forming minerals. This is primarily due to
the dominant in
uence of porosity over mineralogy. Com-
monly, porous rocks also have a greater range of density
than those not having signi
cant porosity.
Although density is again not diagnostic of lithology, refer-
ence to
Fig. 3.30
shows some general statements can be made:
In general, carbonates are denser than siliciclastics.
Evaporites are some of the lowest density species owing
to the low density of their constituents, but the large
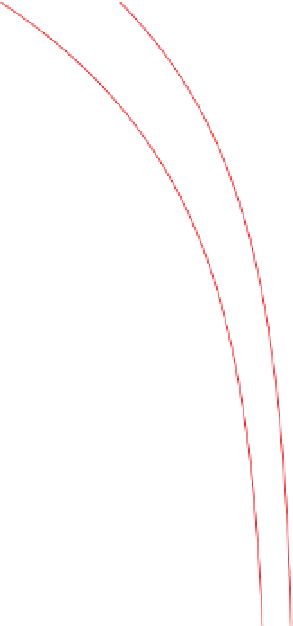




















































































































































































































































































Search WWH ::

Custom Search