Geoscience Reference
In-Depth Information
aftershocks with an angle of 50
is approximately distributed on a velocity boundary at
shallow and moderately depths (E1 and E2 in
Figure 9.7b
)
. The surface extension of this
Near-surface thin layers with significantly low
V
p
(
<
5.0 km/s) and high
V
p
/
V
s
(
>
1.9)
were imaged to the northwest of the mainshock epicenter (X
<
°
0 km). This slow layer
corresponds to the sediments that have piled over the half-grabens formed by crustal
stretching at the time of expansion of the Japan Sea. Since the thickness of the sediments
is less than approximately 3 km, the extent of the crustal stretching is considered to be less
significant compared with the Niigata region
(
Figure 9.2b
)
.
Geological studies show that the crustal shortening that began in the late Miocene (e.g.,
that formed as a normal fault under an extensional stress field. The SE-dipping fault plane
of the mainshock with high dip angle for a thrust-type event is unfavorably oriented in the
direction of the regional maximum stress, which is almost horizontally compressional to the
fault plane is mechanically weak. Therefore, a likely explanation for the Noto-Hanto
earthquake involves reactivation of a normal fault as a reverse fault in terms of inversion
tectonics. The tomographic images associated with the inverted normal fault were also
observed in the source region of the 2004 and 2007 Niigata earthquakes, as mentioned in
the previous sections.
9.4.2 Crustal fluid beneath the mainshock hypocenter
Interestingly, the dip angle of the aftershock alignment changes markedly from 55
°
to nearly
90
0km(
Figure 9.7b
)
. In
addition, the epicenter distributions of these aftershocks beneath the mainshock hypocenter
were linearly concentrated along the fault strike of N55
°
beneath the mainshock hypocenter in the cross-section of Y
=
°
E
(
Figure 9.7a
)
and the size of
the vertical aftershock alignment was approximately 3 km
3 km. Interestingly, both the
mainshock hypocenter and the vertical alignment of aftershocks beneath it are located in
the relatively low-
V
p
zones where the low-
V
p
/
V
s
values were imaged. According to Takei
(
2002
)
, the relatively low
V
p
/
V
s
and low
V
p
can be explained by the presence of water-filled
pores with high aspect ratios.The study of the stress field using high-quality aftershock data
showed that aftershocks that occurred above 4 km in depth indicated a strike-slip stress
indicated a thrust-faulting stress regime. In addition to this depth variation of the stress field,
the maximum principal stress (
σ
1
) axis was stably oriented approximately W20
×
Ndown
to the depth of the mainshock hypocenter, largely in agreement with the regional stress
field, but below that depth the
σ
1
axis had no definite orientation, indicating horizontally
isotropic stress. One likely cause of these drastic changes in the stress regime with depth
is the buoyant force of a fluid reservoir localized beneath the seismogenic zone. Indeed,
the detection of a conductive layer beneath the mainshock hypocenter by a magnetotelluric
°
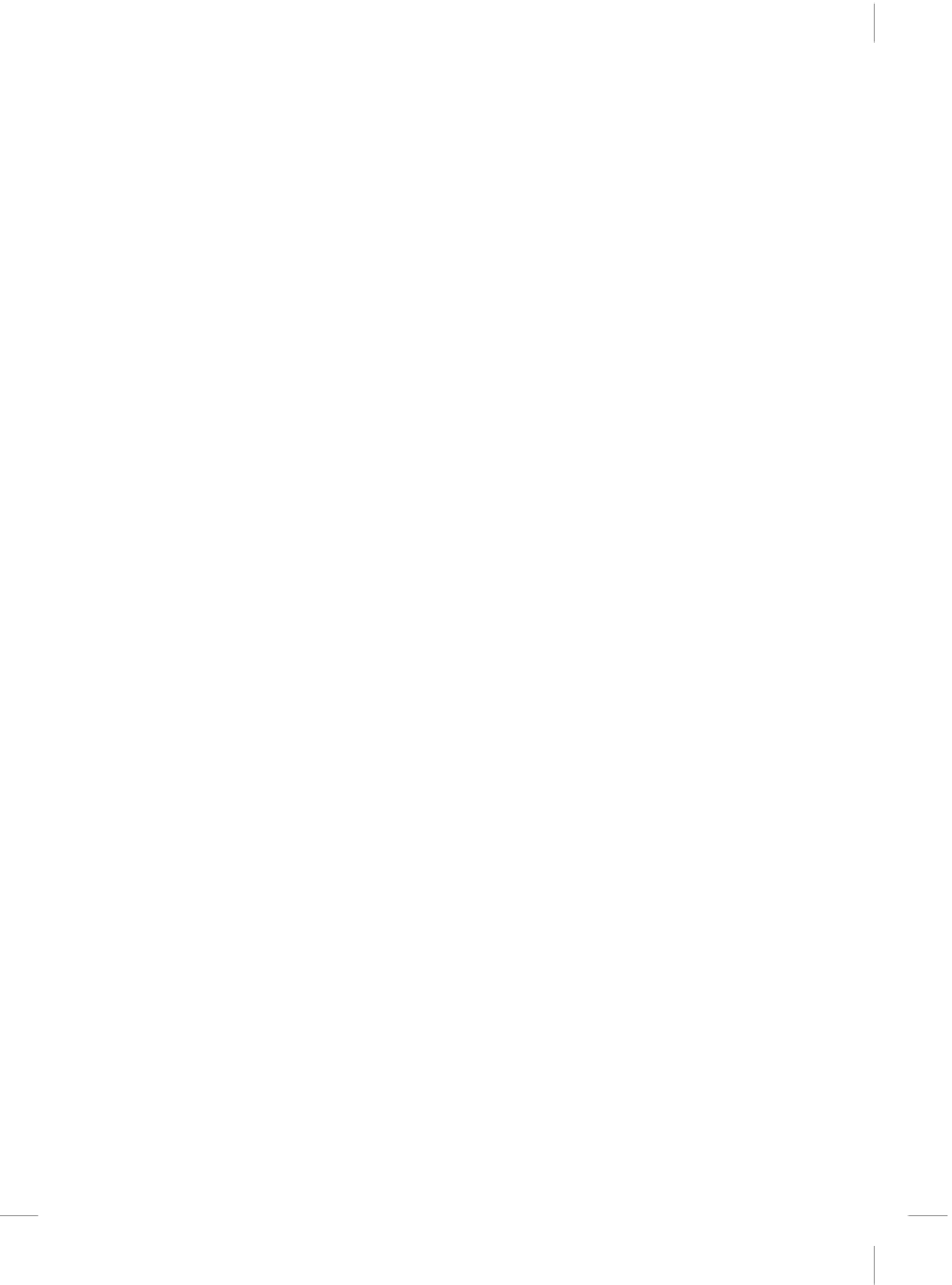