Geoscience Reference
In-Depth Information
the redox conditions. If one assumes that the
Fe
3
+
/
Fe
2
+
ratio does not change during mantle
convection and remains approximately constant
throughout the mantle, there will still be a ma-
jor change of oxygen fugacity with depth, with
the lower parts of the mantle being much more
reducing than the upper parts (Frost & McCam-
mon, 2008). This is mainly for two reasons: (1)
The molar volume of Fe
3
+
is smaller than for
Fe
2
+
, which means that high pressure tends to
shift the equilibrium towards Fe
3
+
. This means
that the same Fe
3
+
/
Fe
2
+
ratio at higher pressures
corresponds to a lower oxygen fugacity. (2) While
olivine dissolves almost no Fe
3
+
, minerals such as
wadsleyite, ringwoodite and in particular alumi-
nous MgSiO
3
perowskite can accommodate much
more Fe
3
+
in their structure. This means that at
the same Fe
3
+
concentration, upon conversion of
olivine to one of these high-pressure phases, Fe
3
+
becomes more ''dilute,'' i.e. the activity of Fe
3
+
and therefore the oxygen fugacity decrease. As a
consequence of this, the lower mantle becomes
so reducing that part of the iron is reduced to iron
metal (Frost
et al
., 2004), which coexists with
a perovskite phase enriched in Fe
3
+
. Therefore,
in the lower mantle, oxygen fugacity is expected
to be close to the iron wustite (Fe-FeO) buffer,
while the oxygen fugacity of the uppermost man-
tle is five orders of magnitude higher, close to
the quartz magnetite fayalite buffer. Immedi-
ately after core formation, the oxygen fugacity
of the entire mantle should have been close to the
(Fe-FeO) buffer. However, there is evidence from
Hadean zircons that a more oxidized upper man-
tle was already established very early in Earth's
history (Trail
et al
., 2011).
In the shallow upper mantle, CO
2
-bearing flu-
ids may be the main reservoir of carbon. In a
pressure range of about 2.5-3.5 GPa a series of re-
actions occurs that convert CO
2
into carbonates
(Wyllie & Huang, 1976), particularly dolomite
and at higher pressures magnesite. At sufficiently
high oxygen fugacity, magnesite could be stable
down to the lower mantle. However, the decrease
of oxygen fugacity with depth likely stabilizes di-
amond deeper in the mantle, probably throughout
most of the transition zone. The likely existence
%
70
60
50
40
30
20
10
0
100
200
300
400
500
600
700
Depth, km
Fig. 1.10
Fraction of total mantle carbon that is
dissolved in silicates as a function of depth. The solid
line is for a depleted MORB source, the dotted line for
a fertile peridotite with 1000 ppm C. After Shcheka
et al
. (2006). Reproduced with permission of Elsevier.
As a result of the very low solubility of carbon
in mantle minerals, the behavior of carbon in the
mantle is fundamentally different from water.
While all of the water can be accommodated as
OH point defects in silicates, most of the carbon
must form some accessory phases. Only for a
rather carbon-poor bulk composition and only for
a thin layer just above the 410 km, more than 50%
of the total carbon may be dissolved in silicates
(Figure 1.10).
1.4.2 Carbon-bearing phases in the mantle
Mantle xenoliths contain carbon mostly in the
form of carbonates or as graphite or diamond.
Since these minerals contain carbon in differ-
ent oxidation states, the stable form of carbon
in the mantle cannot be discussed without con-
sidering the oxygen fugacity in the mantle. Very
likely, the redox conditions in the mantle are con-
trolled by the activities of Fe
2
+
and Fe
3
+
, since
iron is the most abundant element that occurs
with two different oxidation states in the mantle.
However, under some circumstances, in a very
carbon-rich mantle source, it may be possible
that the oxidation state of carbon itself controls
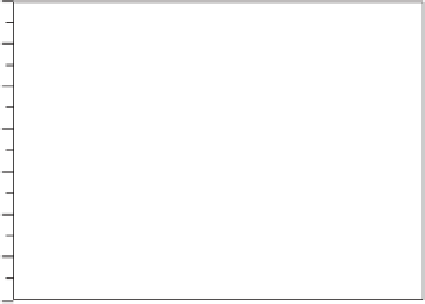
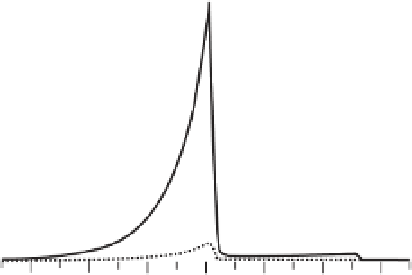