Geoscience Reference
In-Depth Information
(b)
(a)
r
a
r
= Radius
of curvature
(+ve outward)
u
= Mean velocity
A
A
d
r
u
A
B
u
u
B
d
a
B
u
u
(c)
Hydrostatic gradient =
dh
/
dr
D
Change in velocity (negative inward) from
A
to
B
over
centerline distance
dr
is:
−
du
=
u
B
/
u
A
.
Acceleration,
a
, over time taken to travel
dr
is:
a
= -
du
/
dt
.
C
h
2
h
1
At the limit,
a
s
d
t
goes to 0, since angle
a
is common:
−
du
/
u
=
dr
/
r
,
So:
du
= -
udr
/
r
and
a
= -
du
/
dt
= - (
u
/
r
)(
dr
/
dt
).
At the limit, as
dt
goes to 0,
dr
/
dt
= -
u
and
a
= -
u
2
/r
or, since
u
=
D
C
r
)
2
/
r
= -
r
2
v
r,
a
= - (
v
v
Fig. 3.32
(a) and (b) To define the radial acceleration acting in curved flow around channel bends; (c) Superelevation of water on the outside
of any bend and sectional view of helical flow cell within any bend.
Third Law we know this will be opposed by an equal and
opposite centrifugal (outward acting) force. This tends to
push water outward to the outside of the bend, causing a
linear water slope inward and therefore a constant lateral
hydrostatic pressure gradient that balances the mean cen-
trifugal force (Fig. 3.31). Although the
mean
radial force
is hydrostatically balanced, the value of the radial force due
to the faster flowing surface water (see discussion of
boundary layer flow in Section 4.3) exceeds the hydro-
static contribution while that of the slow-moving deeper
water is less. This inequality drives a secondary circulation
of water, outward at the surface and inward at the bottom
(Fig. 3.32c), that spirals around the channel bend and is
responsible for predictable areas of erosion and deposition
as it progresses. The principle of this is familiar to us while
stirring a cup of black or green tea with tea leaves in the
bottom. Visible signs of the force balance involved are the
inward motion to the center of tea leaves in the bottom of
the cup as the flow spirals outward at the surface and down
the sides of the cup toward the cup center point.
3.8
Rotation, vorticity, and Coriolis force
Earth's rotation usually has no obvious influence on
motion, that is, motions closely bound to Earth's surface
by friction, such as walking down the road, traveling by
motorized transport, observing a river or lava flow, and so
on. But experience tells us that rotary motion imparts its
own angular momentum (Section 3.1) to any object, a fact
never forgotten after attempted exit from, or walk onto, a
rotating roundabout platform; in both cases a sharp lateral
push signifies an acceleration arising from a very real force
(there
are
those who doubt the “realness” of the Coriolis
force, referring to it as a “virtual” or “pseudo-force”). At a
larger scale, the path of slow-moving ocean currents and
air masses are significantly and systematically deflected by
motion on rotating Earth. Such motions have come under
the influence of the
Coriolis force
, a physical effect caused
by gradients in angular momentum.
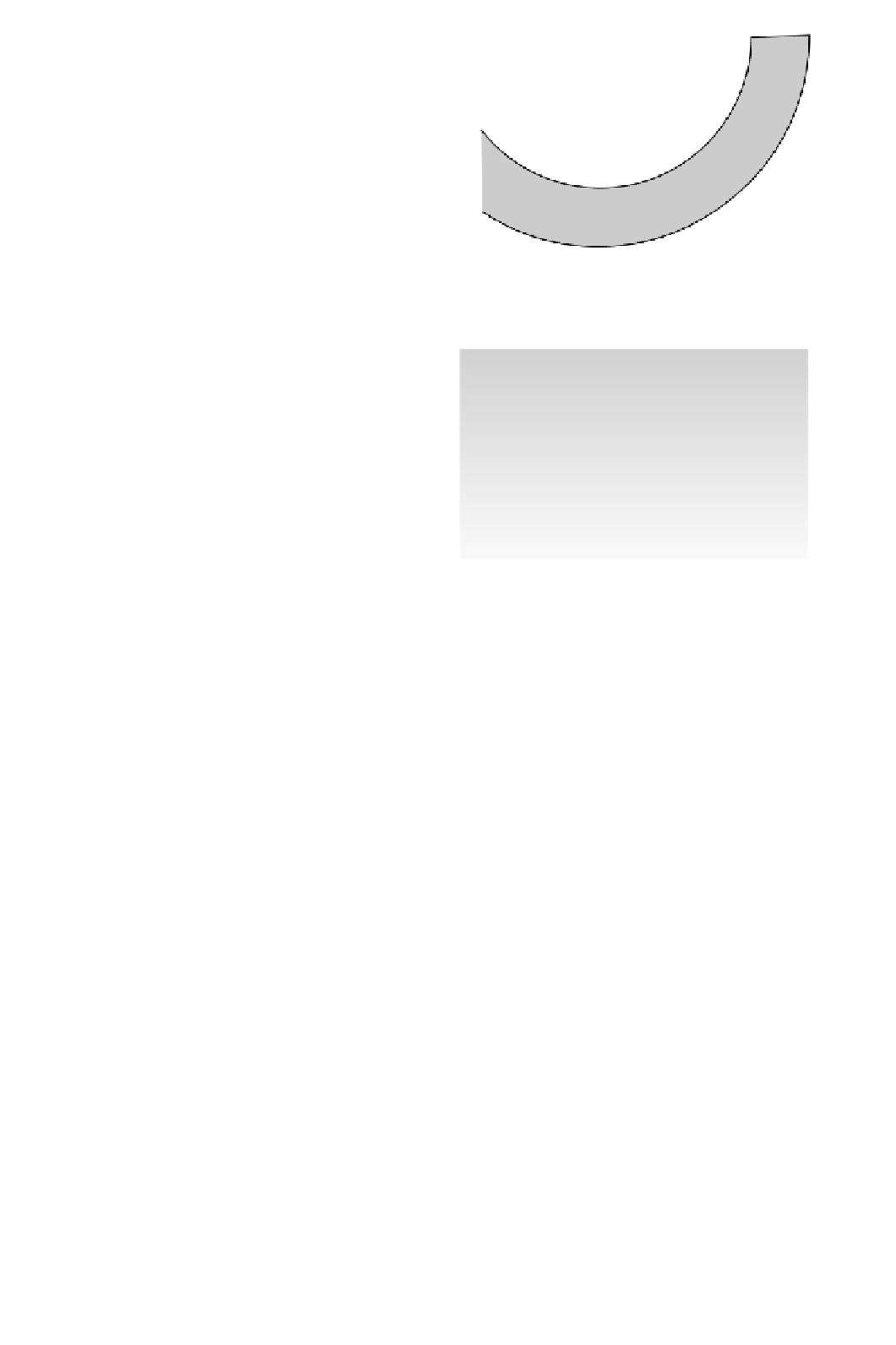




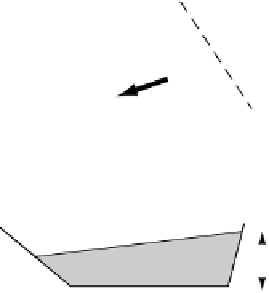






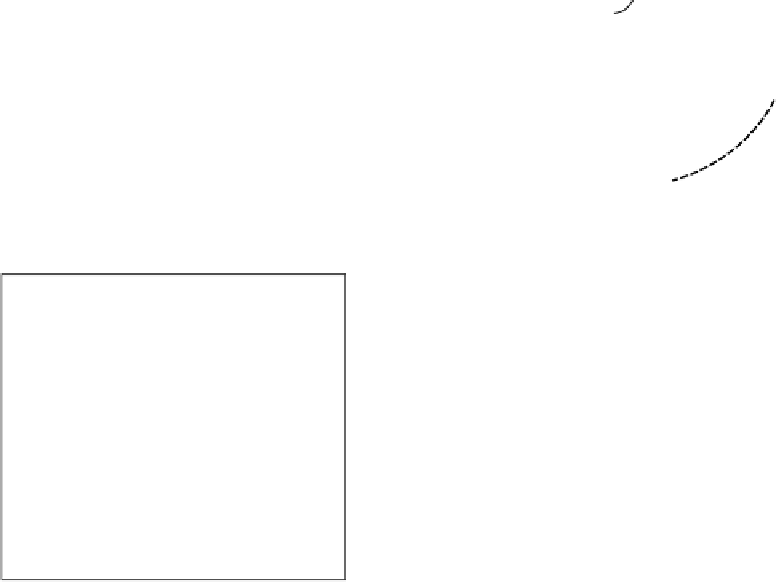
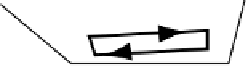
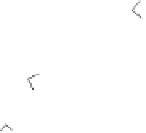





Search WWH ::

Custom Search