Geoscience Reference
In-Depth Information
have periods relating to the Coriolis force and thus are a
strong function of increasing latitude.
In both cases it is the property of vertical propagation of
the internal waves that makes them so effective in spread-
ing momentum; unlike surface ocean waves which only
propagate horizontally. The internal waves cause vertical
internal shear as (d
u
/d
z
)
2
along their wavy interface (cf.
Prandtl's mixing layer theory for turbulent shear flows;
Cookie 12) and it is postulated that such shear zones act as
in any turbulent boundary layer to transfer turbulent
kinetic energy to shorter period eddies as the waves pro-
gressively break up. The mixing process is much more
effective at higher rates of shear and thus the resultant
mixing is more efficacious at higher latitudes where the
Coriolis force,
f
, is greatest.
Fig. 6.29
The general ocean bottom (darker shading) and surface
return legs of the global thermohaline system. Both surface and
deep currents show periodic breakup into spectacular rotating
warm-core eddies, shown here for the surface north Brazilian and
Gulf Stream currents and the deep thermohaline North Atlantic
Deepwater in the South Atlantic.
6.4.5 Benthic oceanic boundary layer: Deep ocean
currents and circulation
We have seen that motion of the upper ocean reflects
momentum exchange across the atmosphere-ocean
interface as modified by vorticity gradients from equator
to pole. But what of the deeper ocean? We still know very
little of the benthic oceanic boundary layer, as problems of
logistics and instrumentation have prevented progress in
the area until quite recently. Radioisotope tracers indicate
that all deep waters must reestablish contact with the
atmosphere on a 500 year timescale. This requires a system
of circulation that allows such links. In the last 40 years,
theoretical results and detailed temperature, density, and
isotopic studies worldwide have revealed a system of deep
(1500-4000 m), dense currents (Fig. 6.29), termed
ther-
mohaline currents
from the dual role that temperature and
salinity have in producing them. Thus at low latitudes the
upper ocean is heated by solar radiation (density
decreases), but also loses water by evaporation (density
increases). At high latitudes the upper ocean is cooled by
contact with a very cold lower atmosphere during winter
(density increases), but freshened by precipitation, river
runoff, and inflows of polar glacial meltwater (density
decreases). At the same time the production of sea ice
leads to saltier residual seawater (density increases).
Thermohaline circulation can thus have several causes,
most varying seasonally, favored by destabilizing processes
that lead to density inversions due to increased surface
water density and the production of negative buoyancy.
There is also a vital role played in cold water formation by
atmospheric wind forcing and Ekman suction/pumping
(Section 6.2), chiefly by regional gyres of high vorticity
like the Irminger Sea tip jet to the east of Greenland
S
S
17 Sv
Fig. 6.30
Cold water sources and generalized flow of North Atlantic
deepwater (
T
C). S - major sources of downwelling in the
Labrador and Greeenland seas, the latter due to wind shear by the
Irminger tip jet.
1.8-4
(Fig. 6.30), and by more local shear producing mixing
gyres, as in the
mistral
wind in the West Mediterranean
and the
bora
of the Adriatic.
Thermohaline currents are linked to compensatory
intermediate and shallow warmer currents in a compli-
cated pattern of downwelling and upwelling, whose
detailed paths in the Pacific and Indian Oceans are still
uncertain. The amount of water discharged by the cur-
rents is staggering, one estimate for deepwater being some
5 ยท 10
7
m
3
s
1
(50
Sv
[
Sverdrup units
: each 10
6
m
3
s
1
]).
This is about 50 times the flow of the world's rivers; about
half of the total ocean volume is sourced from the cooled
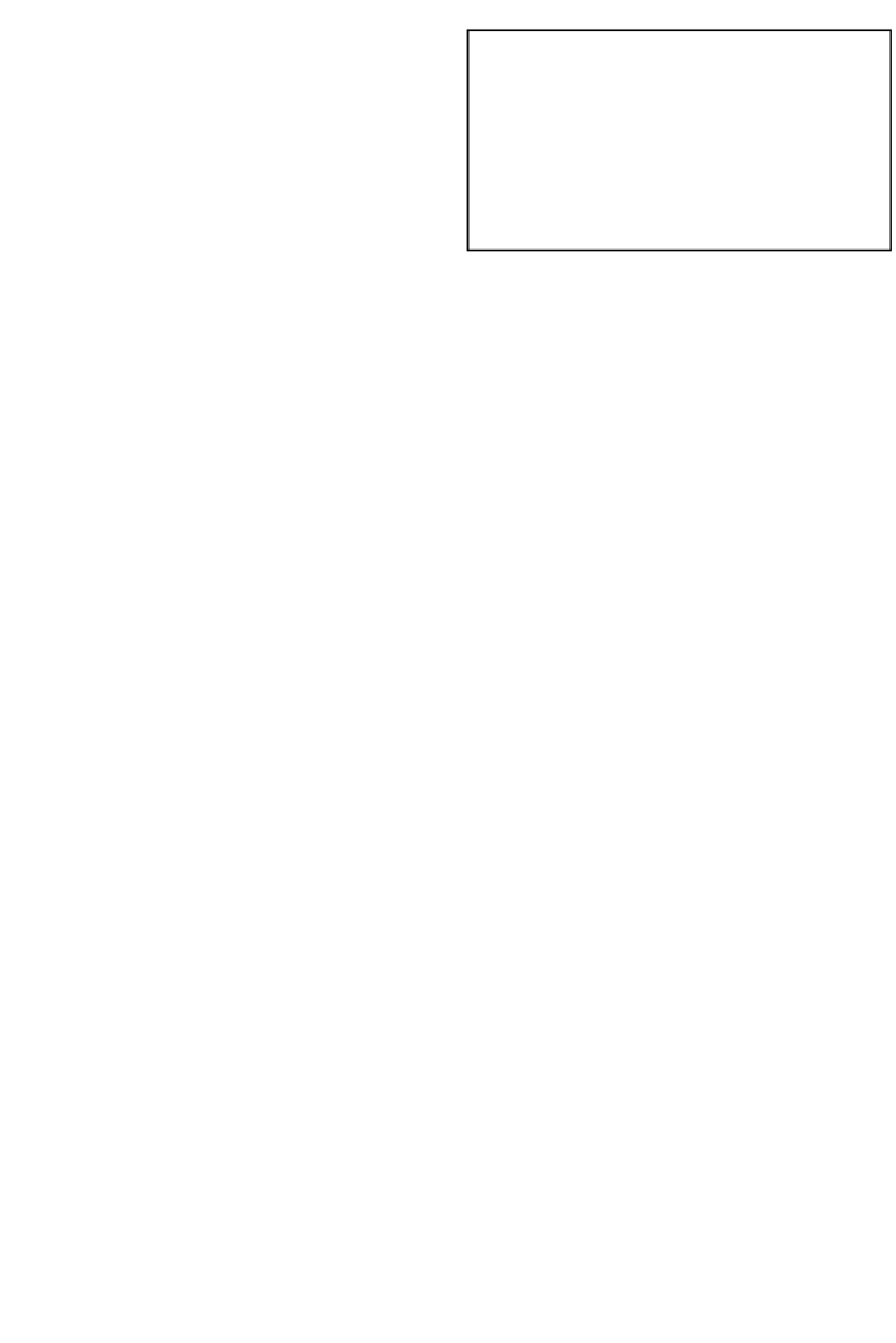


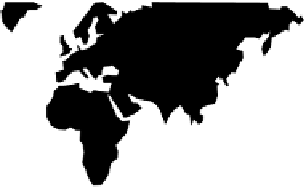




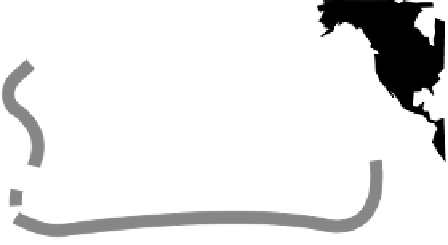




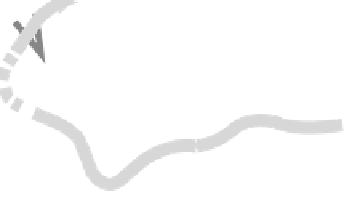




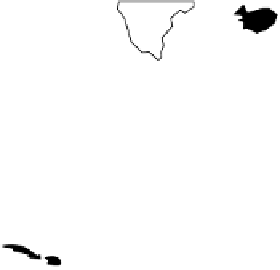
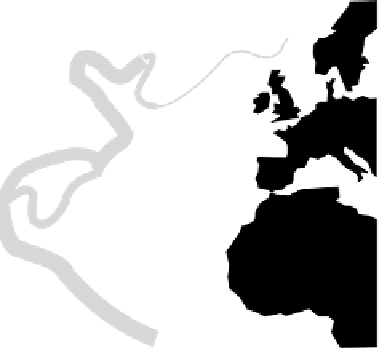




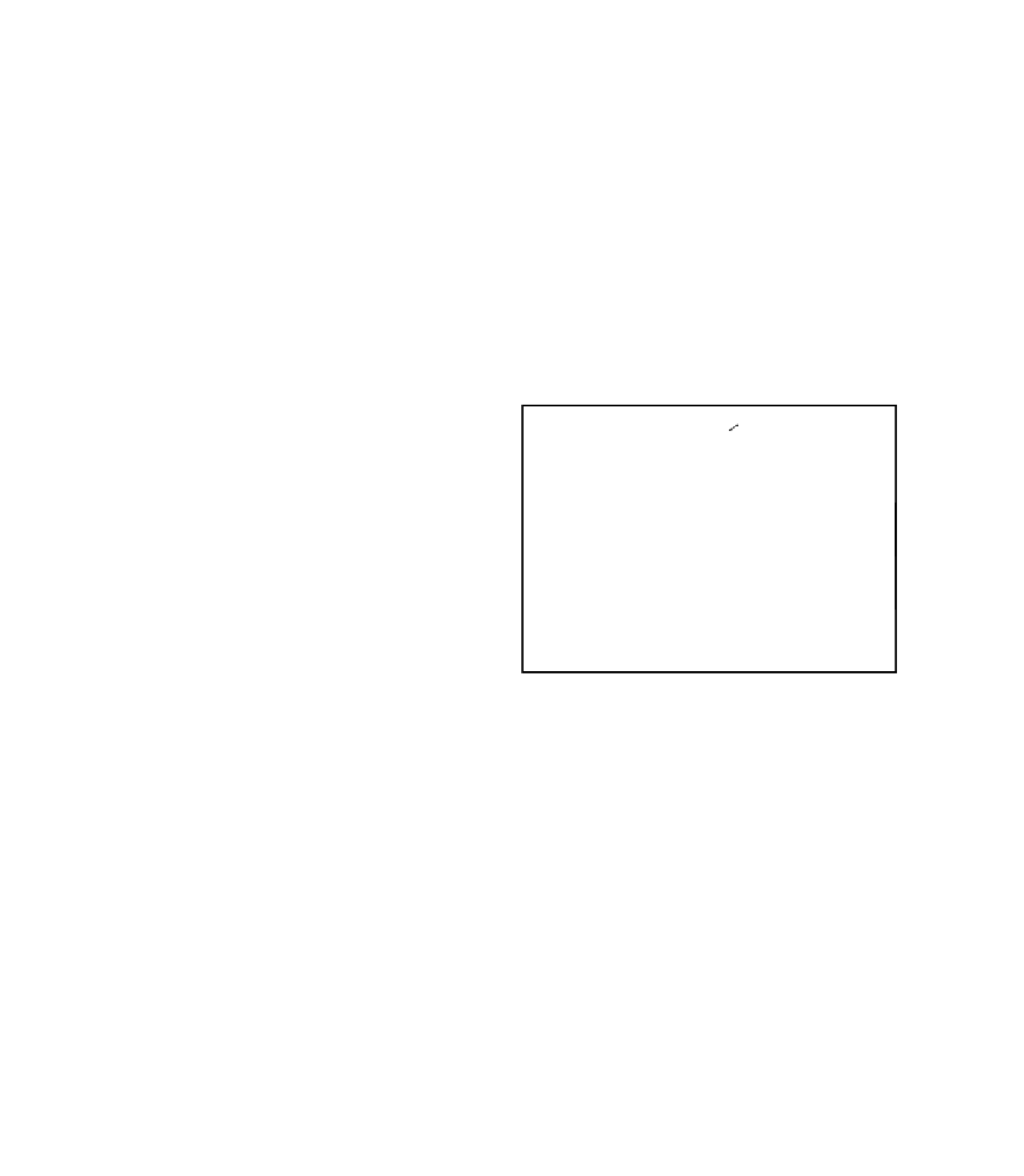
Search WWH ::

Custom Search