Geoscience Reference
In-Depth Information
and friction coefficients for viscous flow through the
crack-conduit system. Discharge rate and the discharge
“magmograph,” or time-series, will drive the subsequent
course of the eruption cycle. For given discharge rates and
duration, the velocity, runout length, aspect ratio (flow
thickness : runout length ratio), and morphology are
closely related to viscosity and development of non-
Newtonian rheologies.The latter exert a major control on
flow behavior through the magnitude of
flow yield strength
(Section 3.15), which must be overcome if flow is to con-
tinue; it most obviously manifests in the presence of well-
developed lateral
levees
and overhanging flow snouts
(Figs 5.2, 5.27, and 5.28). The viscosity control of flow
morphology reaches its apotheosis in acid melts of rhy-
olitic composition. Here viscosity is so large and the effu-
sion temperature relatively low that the melts erupt and
flow very slowly and for short distances as bulbous
rhyolite
domes
(Fig. 5.15) and
rhyolite fans
of high aspect ratio; the
rapidly cooled outer glassy shells are fed from within and
expand like surrealistic onions. The rapid cooling and
glassy texture make the internal planes of viscous shear
clearly visible in ancient examples as
flow banding
.
Rapid quenching is also the most morphologically
distinctive feature of all lava flows, the
pillow lavas
of
subaqueous eruptions. These are tube-like features,
perhaps better described as “bolster lavas” when seen in
3D and is formed as basalt lava extrudes like toothpaste
through the glassy carapaces of previous bolsters. We may
regard the forms simply as extreme examples of
viscous
fingering
, the result of
Saffman-Taylor instabilities
at the
interface of extruding and ambient fluids of contrasting
viscosity (Fig. 5.25). At the midocean ridges and in the
ocean basins where hydrostatic pressures are very high
(25 MPa at 2.5 km depth) there is very little obvious asso-
ciated devolatilization, since sparse exsolving gases cannot
enlarge into significant numbers (
flows is thought to rise through the flows, lubricating their
travel and forming lava pillars. The steam accumulates in
cavities below upper chilled
glass rims
that later collapse
into fragments as the vapor phase condenses. Such brec-
ciated lobate flows, pillows, and bolsters form accumula-
tions many hundreds of meters thick, thus defining the
outer layer of igneous ocean crust.
Explosive eruptions
(Figs 5.29 and 5.30) are favored by
melts with (1) high content of volatile phases, chiefly water
(up to a few wt%) and CO
2
, (2) high viscosity, and (3)
shallow level contact with surface water. The combination
of the first and the second leads to
magmatic explosions
,
which occur frequently in volcanic arcs where, as we have
seen, partial melting of mantle wedge peridotite is permitted
by copious dewatering of serpentinite above subducting
slabs. The eruption products of such explosions tend to be
dominated by magmatic materials. The third process leads
to
phreatomagmatic explosions
, which occur when any
magma type has high-level contact with abundant near-
surface (
phreatic
- Section 6.7) waters. The explosive
energy transformation involved can be very efficient in the
subsurface; eruption products include shattered ambient
rock (called
country rock
by geologists) mixed up with the
more abundant primary magmatic products.
Magmatic explosive eruptions are marked by the produc-
tion of vertical
eruption columns
(Figs 5.30-5.33) of mixed-
size particulate and gas phases. Initially these
free jets
are
pushed skywards at a certain muzzle velocity (of order
10
2
-10
3
ms
1
) by the initial effects of volcanic gas
5 vol.%) of visible cav-
ities or
vesicles
. However, steam produced from seawater
boiling (above 400
C at 30 MPa) below advancing lava
Fig. 5.27
Channelized flowing basalt a 'a ' lava flow, Mauna Loa,
Hawaii 30/3/84. Note older vent and onlapping lava plains
(dark).
Fig. 5.28
Flow of basaltic pahoehoe lava across road, Kilauea,
Hawaii, 16/7/90.

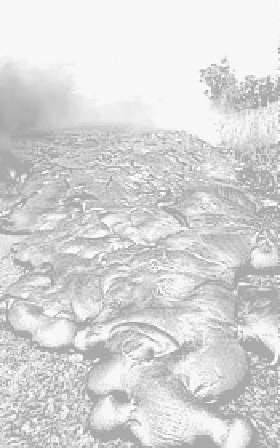
Search WWH ::

Custom Search