Geoscience Reference
In-Depth Information
a well-defined boundary against an outer shell. Approximate
wave speeds appropriate to travel in the Earth's mantle
computed from Oldham's data are tabulated in Fig. 4.130.
(Section 4.9), surface seismic waves are
dispersive
in that
their speed of travel (
celerity
) depends upon wavelength.
They are divided into two groups, those with a horizontal
to- and fro-oscillation normal to the direction of travel
(
Love waves
) and those with an orbital motion in the direc-
tion of travel (
Rayleigh waves
), the latter resembling the
orbitals observed in ocean water surface layers induced by
waves (Section 1.35). The horizontally vibrating
S
-waves
also share with light waves the property of
polarization
,
the separation of oscillations into vertical (
SV
-waves) and
horizontal (
SH
-waves) planes in this case, generated as the
waves strike internal discontinuity surfaces within Earth.
Complete seismogram wave arrivals are illustrated in
Fig. 4.133. Like light and water waves, seismic waves
also
reflect
and
refract
, observing
Snell's laws
as they do so
(Figs 4.134-4.136).
4.17.2 Modern parlance for seismic waves and
their magnitude
Seismic tremors propagate elastic energy from a natural
rupture source (fault) or human-made explosion as oscilla-
tory 3D motion. The wave-like tremors spread radially as
body
and
surface waves
, losing energy as they spread geo-
metrically outward. The lower amplitude body waves
spread faster along curved paths deeper within Earth
(Fig. 4.131) because of elastic compression. Body waves
are themselves separable (Fig. 4.132) into higher speed
compression-rarefraction waves (termed primary, pressure,
push-pull, or usually just
P-waves
) that physically resemble
sound waves and slower, transversely oscillating waves
(termed secondary, shake, shear, or just
S-waves
). Unlike
P
-waves, the latter cannot travel through fluid, but they
may travel through partially molten solid, slowing down
(attenuating) as they do so. Like deep-water waves
4.17.3 Speeds and types of seismic wave
interactions with internal discontinuities
In order to determine depth to possible internal disconti-
nuities in Earth's interior the velocity of seismic waves
must first be determined. For penetration of acoustic
waves generated by various artificial energy sources
through water, including the well-known sonar, this is no
problem. Experiments in water bodies of known depth
yield the figure for acoustic velocity in seawater of about
1.5 km s
1
, the acoustic wave energy traveling outward
from source as straight rays. However, for the largely
unknown rocks of Earth's interior the pioneers of seismol-
ogy had to make use of what is termed an “inverse prob-
lem” approach, that is, they had to deduce the velocities
from a knowledge of the variation of travel time,
T
, with
distance,
º
P
S
∆
9.2
5.5
30
50
9.3
5.3
10.4
6.5
70
11.1
6.9
90
R.D. Oldham
1
º
arc =
111 km
km s
-1
). We have seen already that such
travel time plots can be generated and that for
P
- and
, since
T
f
(
Note 10 min delay
50
40
2nd phases
(
S
-waves)
B
Delays and
scatter
30
D
i
B
A
i
D
20
R
1st phase
(
r
D
10
P
-waves)
R
0
∆
, Distance (degrees of arc from earthquake source)
30
60
90
120
150
180
∆
C
Fig. 4.130
Summary of data Oldham used to “x-ray” Earth. Note the
delays and scatter of
P
-waves recorded at large arc distances and
stepped slowdown of
S
-waves beyond about 130
Travel time,
T
, varies with the angle
∆
,
so that the ray parameter,
p
= d
T
/d
∆.
arc: both indicative
to Oldham of a central dense core, later shown to be liquid. The dis-
tant
S
-waves are now known to be reflected mantle
S
-waves, the direct
waves not traveling through the liquid core. Note
Fig. 4.131
For any curved ray like
AB
, speed,
V
, varies along the
path. For any point, D, of a ray Snell's Law defines a constant ray
parameters,
p
, such that
r
D
/
V
D
R
sin
i
B
/V
B
velocities with
.
p
.

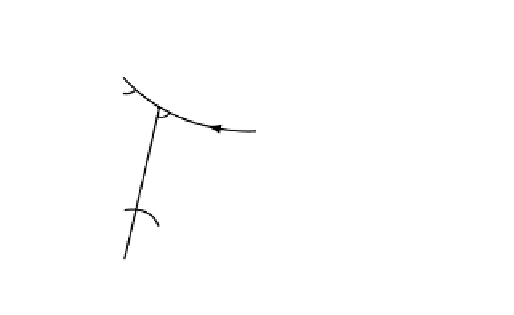
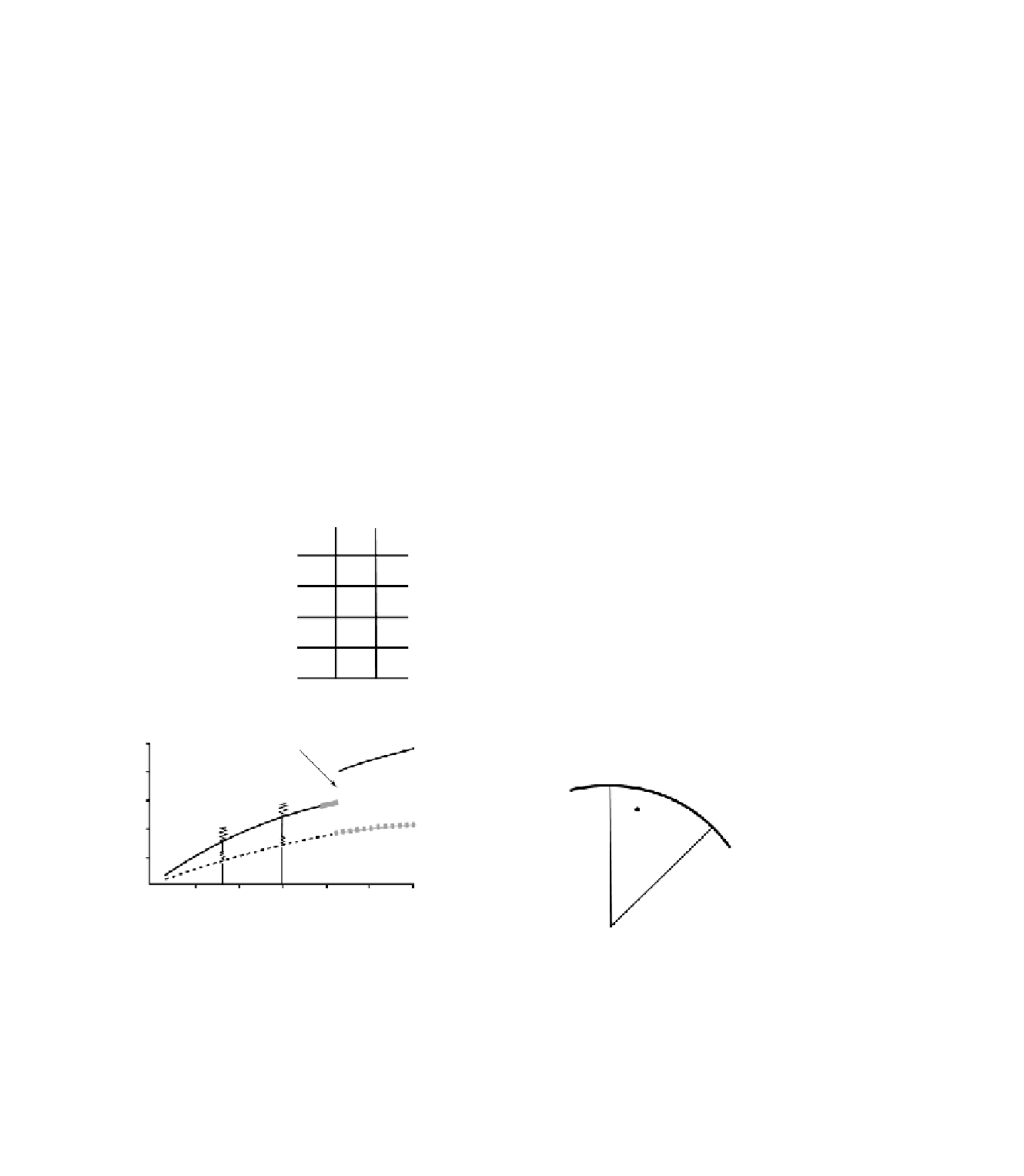
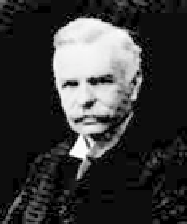
Search WWH ::

Custom Search