Geoscience Reference
In-Depth Information
L
L
H
H
Streamlines for fluid motion
wrt small rising bubble
Re
g
for large bubble = c x 10
-3
Fig. 4.33
Two air bubbles are rising through syrup. The larger is moving faster and catches up with the smaller. As the larger encroaches into
the low velocity/high pressure field created below the smaller it is strained by extension into a prolate ellipse. The smaller is strained by its own
pressure field due to motion, with a lateral flattening due to decreased spanwise pressure about its equatorial plane. Eventually the bubbles
collide and coalesce, the smaller spreading over the larger.
(Fig. 4.31) the drag increases more rapidly and Stokes
equation no longer applies. The periodic eddy motions
shed off alternate sides of a settling particle during viscous
and turbulent flow separation are known as
von Karman
vortices
. In multi-particle settling the fluid streamlines of
individual grains interact. Increased drag results in a
decrease of fall velocity (Box 4.1).
eruptions (Section 5.1). Stokes law can be used to predict
the rate of ascent of the bubbles, though bubble deforma-
tion and independent controls on the rate of bubble
growth during motion make the analysis a good deal more
complicated than for simple solid motion. The motion of
multiple bubbles involves interaction between velocity and
pressure fields; this imposes strain upon the moving and
interacting bubbles (Fig. 4.33). The visible straining of
bubbles makes it clear that the motion of multiple solid
particles, be they moving through still fluid or in a shear
flow, distort the flow pressure field and exert additional
pressure forces on distant flow boundaries. These affects
are important in the movement and size segregation of
all particles in granular and fluid-granular mixtures
(Section 4.11).
4.7.3 Bubble motion and mutual particle
interactions
The movement and deformation of growing gas bubbles
in cooling, flowing magma is an important process in
magmatic intrusions (see Fig. 4.17) and during volcanic
4.8
Particle transport by flows
Natural fluid flows often interact with a solid boundary. If
the boundary comprises sediment particles these can inter-
act with the fluid boundary layer. The study of fluid-
sediment interactions is termed
loose boundary hydraulics
.
being exchanged between one mode of transport and the
other.
Particles initially at rest under a turbulent boundary
layer are subject to both shear (drag) and lift forces
(Fig. 4.35). The shear force arises from gradients of vis-
cous or turbulent shear stress in the flow boundary layer
(Sections 4.3-4.5), while the lift force arises from the
Bernoulli effect (Section 3.12). Both shear and lift forces
act on the protruding parts of particles resting on the
boundary. When the combined magnitude of these is suf-
ficient to overcome particle inertia and friction then the
particles will move. In practice (Fig. 4.36), because of vari-
able bed particle size and particle exposure to turbulent
fluctuations, the threshold condition for initiation of motion
must be determined experimentally and expressed using
dimensionless numbers (Fig. 4.37): threshold conditions
4.8.1
Initiation and paths of grain motion
It is conventional to separate transported sediment in
regular, if intermittent, contact with the bed from that
traveling aloft in the flow. The former is known as
bed
load
, the latter as
suspended load
. A turbulent fluid shear
stress,
zz
,
drives the latter (Fig. 4.34; Cookie 13). For individual
particles this division of sediment transport is often a
statistical one, since individual particles are constantly
zx
, drives the former, while a normal stress,
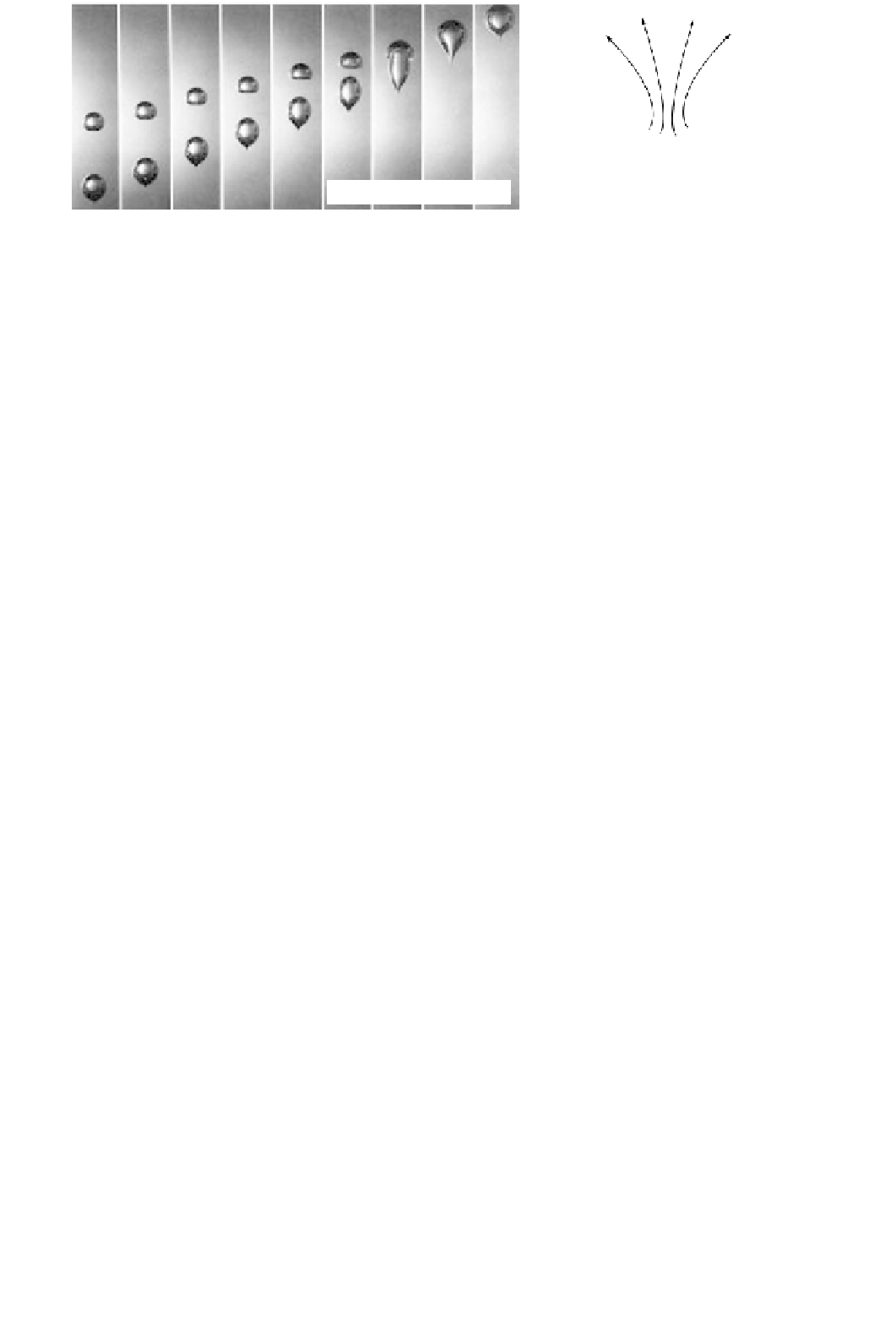

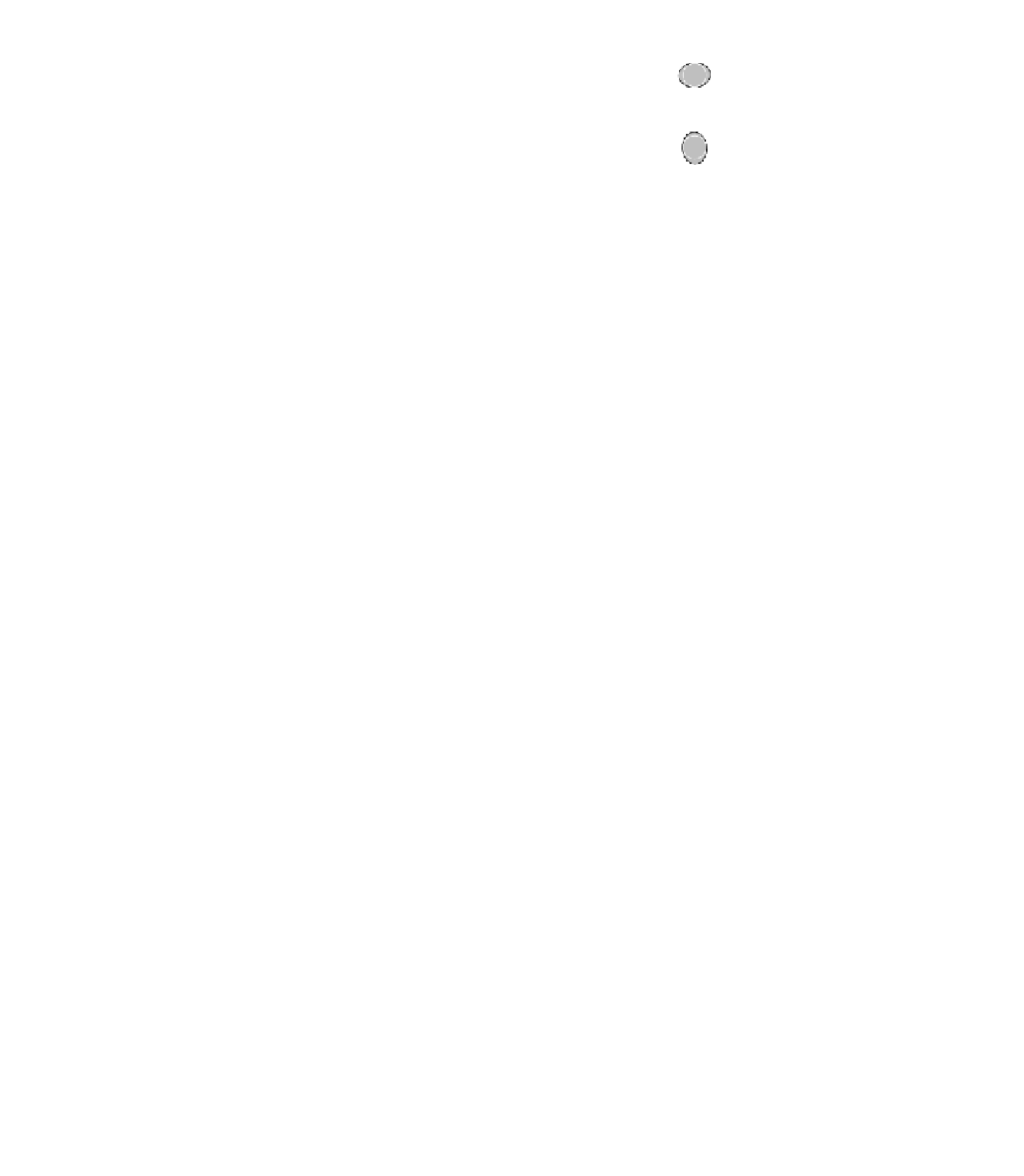
Search WWH ::

Custom Search